Transition Metal Catalysis: Exploring Applications in Inorganic Chemistry and Assignments
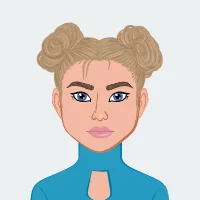
Transition metal catalysis is a fundamental pillar in the realm of inorganic chemistry, serving as a catalyst for progress in various applications. This blog post seeks to demystify the intricate domain of transition metal catalysis, navigating through its foundational principles to its state-of-the-art applications. The journey will extend beyond theoretical understanding, exploring practical assignments that shed light on its paramount role in both academic and industrial landscapes. Whether you require help with your inorganic chemistry assignment or seek to delve deeper into the realm of transition metal catalysis, this blog provides valuable insights and perspectives to enrich your understanding and proficiency in this essential area of chemistry.
At its core, transition metal catalysis involves the use of transition metals, such as iron, copper, and ruthenium, to facilitate chemical reactions. These metals possess unique electronic configurations that make them adept at participating in catalytic processes, influencing reaction pathways and accelerating reaction rates. Understanding the principles governing these reactions is crucial for comprehending the intricacies of transition metal catalysis.
The significance of transition metal catalysis reverberates across diverse applications. From pharmaceuticals to materials science, transition metal catalysis has enabled breakthroughs in drug synthesis, polymerization reactions, and the creation of advanced materials. By manipulating the electronic and steric properties of transition metal complexes, chemists can tailor catalytic systems for specific applications, opening avenues for innovation.
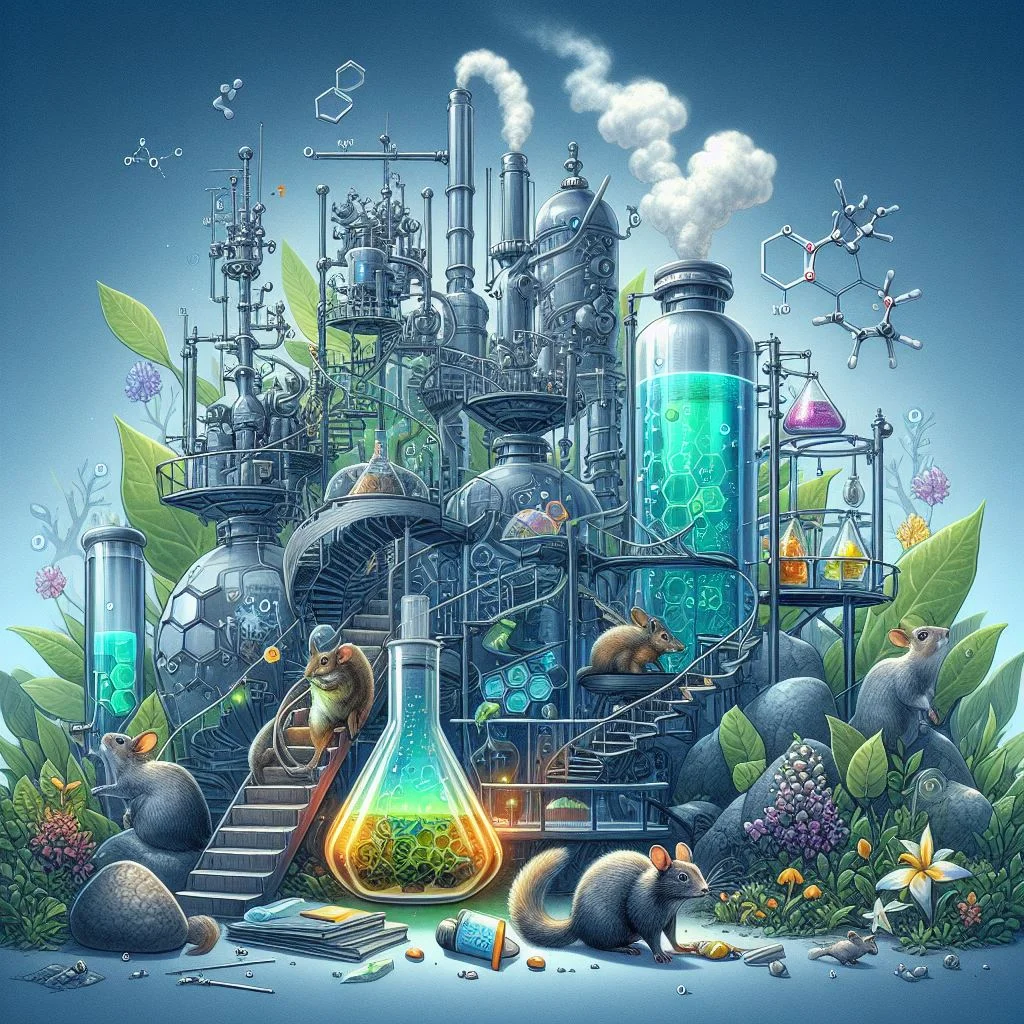
In the academic realm, students delve into the world of transition metal catalysis through theoretical studies and hands-on experiments. These practical assignments not only reinforce theoretical knowledge but also instill a deeper appreciation for the complexities of catalytic processes. As students design and optimize catalytic systems, they develop critical thinking and problem-solving skills essential for a career in chemistry.
Transition metal catalysis is not confined to the laboratory; its impact extends to industrial settings. Catalytic processes utilizing transition metals play a pivotal role in the synthesis of bulk chemicals, pharmaceuticals, and fine chemicals on an industrial scale. The efficiency and selectivity of these catalytic systems contribute to sustainable and economically viable manufacturing processes.
Understanding Transition Metal Catalysis
Transition metals, positioned strategically in the central region of the periodic table, emerge as catalysts extraordinaire due to their distinctive properties that set them apart. Their remarkable ability to undergo multiple oxidation states and form stable complexes with ligands renders them invaluable in catalyzing a diverse array of chemical reactions. At the heart of their catalytic prowess lies the involvement of d-orbitals, which play a crucial role in the formation of coordination compounds.
One of the defining features of transition metals is their capacity to exist in various oxidation states, a characteristic stemming from the incomplete filling of their d-orbitals. This flexibility allows them to readily participate in redox reactions, where electrons are transferred between reactants. The ability to shuttle between different oxidation states enables transition metals to act as electron carriers, facilitating reactions that involve electron transfer, such as those in biological processes.
Transition metals' knack for forming stable complexes with ligands further amplifies their catalytic capabilities. Ligands are molecules or ions that can donate electron pairs to the metal, forming coordination compounds. This unique bonding ability enhances the reactivity and selectivity of transition metals in catalyzing chemical transformations. The stability of these complexes ensures a long-lasting and effective catalytic performance.
The d-orbitals of transition metals play a pivotal role in their catalytic activities. These orbitals enable the formation of coordination bonds with ligands, creating a spatial arrangement that facilitates reaction pathways. The availability of d-electrons allows transition metals to undergo electron transfer easily, contributing to their efficacy as catalysts in redox reactions.
Homogeneous and Heterogeneous Catalysis
Transition metal catalysis encompasses two main modalities: homogeneous and heterogeneous catalysis, each contributing significantly to diverse chemical processes. In homogeneous catalysis, the catalyst and reactants coexist in the same phase, exemplified by transition metal complexes. These complexes play an active role in reactions like hydrogenation, oxidation, and isomerization, showcasing their efficacy in manipulating molecular transformations.
Conversely, heterogeneous catalysis involves catalysts existing in a distinct phase from the reactants. Here, transition metal nanoparticles or surfaces act as catalytic agents, facilitating reactions at solid-liquid or solid-gas interfaces. This versatility finds application in various industrial processes, demonstrating the adaptability of transition metals in shaping catalytic pathways.
Homogeneous and heterogeneous catalysis offer unique advantages. The former allows for precise control over reaction conditions due to the well-defined nature of molecular catalysts, while the latter provides practical benefits such as easier catalyst recovery and reuse. Transition metal catalysis, in both forms, stands as a cornerstone in modern chemistry, driving advancements in synthetic methodologies and contributing to the development of novel materials and pharmaceuticals. The synergy between homogeneous and heterogeneous catalysis, mediated by transition metals, underscores their pivotal role in the molecular orchestration of chemical transformations.
Applications of Transition Metal Catalysis
In the realm of chemistry, organic synthesis stands as a cornerstone for the creation of intricate molecules, and transition metal catalysts emerge as indispensable tools in this process. These catalysts, with their unique ability to facilitate chemical reactions, contribute to the construction of complex structures with unparalleled precision. Among the various techniques, cross-coupling reactions, a hallmark of transition metal catalysis, play a pivotal role. These reactions allow the seamless linkage of different organic fragments, unlocking pathways for the synthesis of pharmaceuticals, agrochemicals, and advanced materials.
Transition metal catalysts act as molecular architects, guiding reactions to form specific bonds and configurations. This precision is particularly crucial in drug development and materials science, where the exact arrangement of atoms can significantly impact functionality. The synthesis of pharmaceuticals, for instance, relies on the controlled assembly of molecular components, and transition metal catalysis provides the finesse required for such intricate processes.
Environmental Remediation: A Catalyst for Sustainability. Transition metal catalysis extends beyond the confines of the laboratory, finding application in environmental remediation efforts. These catalysts serve as catalysts for sustainability, aiding in the reduction of environmental impact through various applications. One notable example is the use of transition metal catalysts in catalytic converters in automobiles. These converters facilitate the conversion of harmful emissions into less harmful substances, contributing to cleaner air and mitigating the impact of vehicular pollution on the environment.
Additionally, transition metal catalysis plays a crucial role in the decomposition of pollutants in water. Through carefully designed catalytic processes, these metals assist in breaking down and neutralizing harmful substances, promoting water purification and ecosystem preservation.
In essence, transition metal catalysis emerges as a versatile and powerful tool, both in the controlled realm of organic synthesis and the broader arena of environmental sustainability. Its applications span from the intricacies of molecular design to the macroscopic challenges of mitigating environmental pollution, making it an essential component in the pursuit of precision and sustainability in the fields of chemistry and beyond.
Energy Storage and Conversion: Powering the Future
Transition metal catalysis is pivotal in advancing energy storage and conversion technologies, spearheading transformative developments in the field. Catalysts rooted in transition metals play a crucial role in fuel cells, facilitating the conversion of chemical energy into electrical energy. This application is particularly significant in the realm of clean energy, where fuel cells stand as a promising alternative for sustainable power generation.
Moreover, transition metal-based catalysts contribute significantly to the quest for efficient hydrogen production. Hydrogen, recognized as a clean and versatile energy carrier, is central to the pursuit of sustainable energy sources. Transition metal catalysts enable the development of streamlined processes for hydrogen production, enhancing the viability and scalability of hydrogen as a key component in the clean energy landscape.
As the demand for sustainable solutions intensifies, the importance of transition metal catalysis becomes increasingly evident. These catalysts not only drive advancements in fuel cell technology but also play a pivotal role in establishing efficient pathways for hydrogen production. The synergy between transition metals and catalysis is thus at the forefront of fostering innovation and progress in the transition towards cleaner and more sustainable energy practices.
Assignments: Bridging Theory and Practice
To deepen students' understanding of transition metal catalysis, academic assignments provide a practical avenue for reinforcing theoretical knowledge. These assignments are designed to engage students in a hands-on exploration of the diverse applications of transition metal catalysts. One common task involves the creation of catalytic pathways tailored to specific reactions, encouraging students to apply their theoretical knowledge to real-world scenarios. This not only hones their problem-solving skills but also fosters a deeper comprehension of the intricacies involved in catalytic processes.
Moreover, assignments often require students to delve into the detailed analysis of catalytic cycles, unraveling the step-by-step mechanisms underlying these transformative reactions. By undertaking such analytical exercises, students gain insights into the intricate interplay of transition metals and substrates, further solidifying their grasp on the subject matter.
Furthermore, these assignments go beyond the laboratory, prompting students to consider the broader implications of transition metal catalysis. This includes investigating the economic and environmental consequences of utilizing such catalysts across various industries. Such a holistic approach not only prepares students for practical applications but also instills a sense of responsibility regarding the ecological and economic sustainability of catalytic processes. Overall, these academic assignments play a pivotal role in bridging the gap between theoretical knowledge and real-world applications in the realm of transition metal catalysis.
Recent Developments in Transition Metal Catalysis
Advancements in technology have propelled a deeper comprehension of transition metal catalysis, marking a progressive era in this field. Noteworthy developments encompass the innovative design of ligands, aimed at augmenting catalytic activity. This strategic approach seeks to optimize the efficiency of transition metal catalysts, offering a promising avenue for further breakthroughs. Additionally, researchers are delving into the utilization of earth-abundant metals as catalysts, a departure from traditional reliance on scarce resources. This shift not only broadens the scope of catalytic applications but also addresses sustainability concerns.
A pivotal aspect of recent progress is the commitment to developing catalytic processes with minimal environmental impact. This conscientious approach aligns with the global pursuit of eco-friendly technologies, marking a departure from conventional practices that contribute to environmental degradation. The integration of green chemistry principles into transition metal catalysis not only enhances the overall efficiency of processes but also underscores the responsibility of the scientific community toward environmental stewardship.
These breakthroughs collectively propel the continuous evolution of transition metal catalysis as a vibrant and dynamic field of study. The synthesis of novel ligands, exploration of earth-abundant metals, and the emphasis on environmentally benign processes epitomize a concerted effort to shape catalysis into a sustainable and impactful discipline, resonating with the demands of a technologically advanced and environmentally conscious society.
Challenges and Future Directions
In the realm of transition metal catalysis, notable strides have been made, yet persistent challenges underscore the need for continuous exploration. Catalyst deactivation, the quest for selectivity in intricate reactions, and the dependence on precious metals remain hurdles prompting further investigation. Addressing these issues is imperative for advancing catalytic processes in diverse applications.
Future directions in this dynamic field pivot on two crucial axes: sustainability and computational integration. Sustainable catalysts, economically viable and ecologically sound, are at the forefront of research priorities. The quest for alternatives to precious metals, often scarce and expensive, propels scientists toward innovations that balance efficacy with environmental responsibility. Simultaneously, the integration of computational methods emerges as a transformative force. Harnessing the power of algorithms and simulations for catalyst design and optimization promises accelerated discovery and precision in tailoring catalytic systems.
The synergy between sustainable catalyst development and computational prowess heralds a new era in catalysis, offering solutions to current limitations and charting a course towards greener, more efficient chemical processes. As researchers delve into uncharted territories, the landscape of transition metal catalysis is poised for transformative breakthroughs that will shape the future of chemical synthesis.
Conclusion
Transition metal catalysis represents a dynamic and indispensable realm within inorganic chemistry, playing a pivotal role in shaping various applications that have a profound impact on our world. This field extends its influence from the intricacies of organic synthesis to the imperative domain of environmental sustainability, making it a linchpin in the development of a more efficient and eco-friendly chemical landscape.
At its core, transition metal catalysis hinges on the use of transition metals as catalysts, which facilitate and expedite chemical reactions without undergoing substantial changes themselves. This nuanced approach has far-reaching implications, and the applications are both diverse and transformative. From facilitating the creation of complex organic compounds to streamlining industrial processes, transition metal catalysis emerges as a versatile and indispensable tool.
This blog post serves as a comprehensive overview of transition metal catalysis, delving into its fundamental principles, myriad applications, practical assignments, recent advancements, and the challenges that lie ahead. The fundamental principles elucidate the underlying mechanisms that govern these catalytic processes, providing a solid foundation for understanding their intricacies. The discussion on applications spans a spectrum, encompassing the synthesis of pharmaceuticals, agrochemicals, and materials crucial for various industries.
Practical assignments demonstrate the real-world applicability of transition metal catalysis, showcasing its utility in laboratory settings and industrial scenarios. The exploration of recent developments sheds light on the cutting-edge research and innovations within this field, underlining its continuous evolution. However, the journey towards harnessing the full potential of transition metal catalysis is not without its challenges. Future considerations encompass refining catalyst design, improving sustainability metrics, and addressing issues related to scalability.
As researchers and practitioners continue to unlock the latent capabilities of transition metal catalysis, the trajectory toward a more sustainable and efficient chemical world becomes increasingly evident. This journey signifies not only scientific progress but also a commitment to mitigating environmental impact and advancing the frontiers of chemistry for the benefit of society.