Important Concepts and Reactions in Carbohydrate Chemistry Assignments
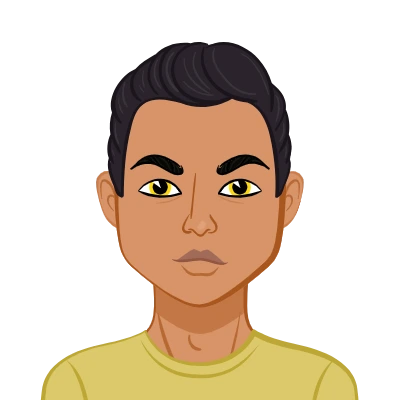
Learn the core ideas and crucial processes involved in carbohydrate chemistry assignments thoroughly. Become familiar with monosaccharides, glycosidic connections, mutarotation, oxidation, reduction, hydrolysis, dehydration, and enzymatic processes. Learn how these fundamental ideas affect carbohydrates' composition, action, and metabolism.
The Main Concepts and Reactions in Carbohydrate Chemistry Assignments
The intriguing topic of carbohydrate chemistry is concerned with the investigation of carbohydrates, vital macromolecules present in all living things. Comprehending the fundamental ideas and processes is essential for students working on carbohydrate chemistry assignments. This blog article will discuss the essential ideas and significant reactions that serve as the cornerstone of carbohydrate chemistry assignment help.
Monosaccharides
The simplest type of carbohydrates, monosaccharides, are used as the building blocks for more complex carbs. They are organic substances made up of just one sugar unit. They are called "mono" because additional hydrolysis to produce smaller sugar molecules is impossible.
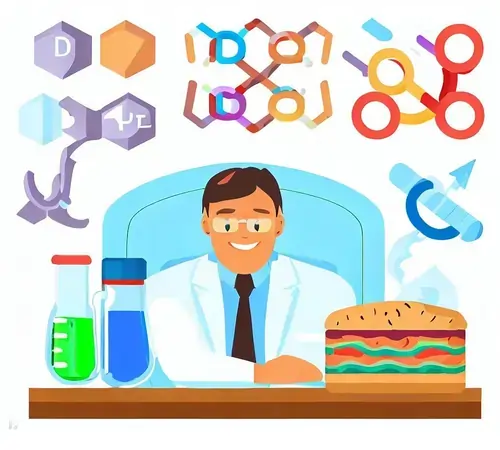
It is important to comprehend the structure of monosaccharides as one of its key characteristics. They frequently have multiple hydroxyl groups (-OH) and either an aldehyde or a ketone functional group, which indicates that they are polyhydroxy aldehydes or ketones. Aldoses, which have an aldehyde group, and ketoses, which have a ketone group, are nature's two most prevalent monosaccharides. A monosaccharide's carbon backbone typically comprises three to seven carbon atoms.
Monosaccharides can also be found as isomers, which are distinct spatial arrangements. Understanding the difference between D- and L- setup is important. These words describe how the hydroxyl group is positioned on the chiral carbon farthest from the carbonyl group. The mirror versions of one another, D and L isomers, each exhibit unique chemical and biological characteristics.
The capacity of monosaccharides to create cyclic structures in solution is another crucial feature. Monosaccharides can go through intramolecular interactions in aqueous conditions that lead to the creation of cyclic hemiacetals or hemiketals. Pyranoses, which have a six-membered ring, and furanoses, which have a five-membered ring, are the two most prevalent cyclic structures.
It is also essential to comprehend how monosaccharides are categorized. Trioses, tetroses, pentoses, and hexoses are examples of the several types of monosaccharides that can be categorized according to the number of carbon atoms they contain. Monosaccharides that are frequently found include glucose, fructose, and ribose.
Numerous biological functions depend heavily on monosaccharides. In addition to participating in glycolysis, cell respiration, and other metabolic pathways, they provide energy. Additionally, they take the role in the synthesis of structural elements such as extracellular matrix molecules and cell membranes.
In conclusion, monosaccharides, which have a basic structure consisting of hydroxyl groups and either an aldehyde or ketone functional group, are the basic building blocks of carbohydrates. Understanding their isomeric forms, cyclic architectures, and classification based on carbon atoms is crucial. To fully understand more complicated carbohydrate structures and how they work in biological systems, one must first understand monosaccharides.
Glucosylated Linkages
In order to build more complex carbohydrates, such as disaccharides, oligosaccharides, and polysaccharides, glycosidic connections are chemical bonds formed between monosaccharide units. These bonds are created through a condensation reaction, also known as dehydration synthesis, in which an oxygen bridge (-O-) is created when a hydroxyl group (-OH) from one monosaccharide combines with the anomeric carbon of another monosaccharide. The glycosidic bond is the name of this oxygen bridge.
For carbohydrates to maintain their structural stability and functional characteristics, glycosidic connections must develop. It controls the position and orientation of monosaccharide units in the carbohydrate chain, affecting the structure, solubility, and biological activity of the chain as a whole. The characteristics and behavior of the carbohydrate molecule can be significantly influenced by the type of glycosidic bond produced.
Glycosidic couplings come in a variety of forms, including - and -glycosidic bonds. These phrases describe how the anomeric carbon, which turns into a hemiacetal or hemiketal carbon in a monosaccharide's cyclic form, is oriented in relation to the oxygen bridge. Anomeric carbon in a -glycosidic linkage faces the -OH group in the opposite direction, but in a -glycosidic linkage, it faces the -OH group in the same direction. The stability and conformation of the glycosidic bond are impacted by this orientation.
The characteristics and activities of the resultant carbohydrate are governed by the particular kind of glycosidic bond that forms between monosaccharides. For instance, a,-1,2-glycosidic bond gives the disaccharide sucrose, which is made up of glucose and fructose, its distinctive sweetness and stability. Contrarily, the disaccharide lactose, which is present in milk, has a,-1,4-glycosidic bond between the sugars glucose and galactose, which impairs its capacity to be degraded by the lactase enzyme.
It is crucial to comprehend glycosidic connections if one is to research the composition and purpose of carbohydrates. Students may be asked to categorize the many types of glycosidic links, anticipate a carbohydrate's properties based on its linkage, or even manufacture carbohydrates with certain glycosidic linkages as part of their carbohydrate chemistry assignments. Therefore, it is essential to have a firm understanding of glycosidic connections in order to solve carbohydrate chemistry puzzles and appreciate the intricacy of carbohydrate structures in biological systems.
Reducing and Non-Reducing Sugars
Important terms in chemistry assignments help define how well some sugars can oxidize including reducing and non-reducing sugars.
Monosaccharides or disaccharides with a free or potentially free aldehyde or ketone group are referred to as reducing sugars. Due to the existence of these functional groups, reducing sugars can interact chemically with Benedict's or Fehling's solution to alter hue. The reagent undergoes reduction while the aldehyde or ketone group undergoes oxidation to a carboxylic acid. The reducing sugars glucose, fructose, and maltose are typical examples.
Non-reducing sugars, on the other hand, are carbohydrates that either don't have a free aldehyde or ketone group or have it in a form that makes it difficult to oxidize. The sugars sucrose, lactose, and trehalose are non-reducing sugars. Due to the lack of the required reactive group, these sugars cannot conduct the normal oxidation reactions with Benedict's or Fehling's solution.
In many physiological and analytical applications, the distinction between reducing and non-reducing sugars is essential. For instance, the ability of glucose, a reducing sugar, to react with particular enzymes or chemical reagents is the basis for the detection of blood glucose levels in clinical diagnostics. The measurement of reducing sugars in food analysis can reveal the degree of carbohydrate breakdown or the presence of certain sugars in a sample.
As cyclic forms can be in equilibrium with the open-chain form, it is significant to highlight that a sugar's reducing or non-reducing nature is tied to its open-chain form. Reducing sugars can also change into non-reducing forms by the production of glycosidic linkages, which unite monosaccharides to produce disaccharides or polysaccharides.
Mutarotation
Mutarotation is a phenomena where the precise rotation of an aqueous solution varies over time and is most frequently seen in certain carbohydrates, especially monosaccharides. This process is brought on by the monosaccharide's interconversion between its two distinct anomeric forms (and). The term "anomeric forms" describes various spatial configurations of the hydroxyl group at the sugar molecule's first carbon, or "anomeric carbon."
A pure monosaccharide initially occurs mostly in one of its anomeric forms (either or) when it is dissolved in water. However, the cyclic ring structure is not completely rigid because of the hemiacetal or hemiketal character of monosaccharides. The free rotation of the hydroxyl group at the anomeric carbon leads to interconversion between the andanomers.
The mutarotation mechanism causes the andanomers to constantly interconvert over time. Because the hydroxyl group can adopt several spatial orientations, a change in the solution's particular rotation results in this interconversion. Temperature, pH, and concentration are a few examples of variables that affect the rate of mutarotation.
Practical applications of the mutarotation technique include the characterization and analysis of carbohydrates. A freshly generated carbohydrate solution first displays a certain rotation that corresponds to the predominate anomer. A weighted average of the particular rotations of the andanomers, known as the equilibrium value, is what the specific rotation eventually reaches, though, over time.
In investigations utilizing polarimetry, a method for determining how optically active materials rotate plane-polarized light, mutarotation is frequently seen. Scientists can precisely study the structure and composition of carbohydrates as well as ascertain the equilibrium combination of anomers present in a solution by comprehending mutarotation.
Oxidation and Reduction Reactions
Fundamental electron-transfer reactions in the chemistry of carbohydrates include oxidation and reduction. The oxidation status of the molecules involved changes throughout these processes. Understanding oxidation and reduction reactions is essential for understanding how carbohydrates behave and change.
A molecule loses electrons during an oxidation reaction, increasing its oxidation state. The oxidizing agent is the molecule that accepts the electrons. An oxidation reaction in the context of carbohydrates frequently involves the transformation of a monosaccharide (like glucose) into an oxidized form. For instance, glucose can be further oxidized to create glucuronic acid or gluconic acid by oxidation. These reactions are frequently utilized in laboratory assessments of the presence of reducing sugars, such as the Tollens test and Fehling's test.
The oxidation state of the molecule is reduced as a result of reduction processes, which include the gain of electrons. The reducing agent is the molecule that provides the electrons. Reduction reactions are frequently seen in the transformation of monosaccharides into sugar alcohols in the study of carbohydrates. For instance, the sugar alcohol known as sorbitol is produced when glucose is reduced. In procedures like the synthesis of complex carbohydrates and the production of energy through carbohydrate metabolism, reduction reactions are also crucial.
In carbohydrate chemistry, oxidation and reduction reactions frequently include one another. For instance, the reduction of an oxidizing agent, such as an oxidizing agent like NAD+, is required for the oxidation of glucose into gluconic acid. Within living organisms, these redox processes are crucial to the breakdown and synthesis of carbohydrates.
Overall, since they provide the basis for numerous transformations and metabolic processes involving carbs, an understanding of oxidation and reduction reactions is crucial in the study of carbohydrates. Students may examine and forecast how carbohydrates will behave in various chemical reactions by understanding these ideas, which will also help them understand more about how they function in biological systems.
Hydrolysis and Dehydration Reactions
The synthesis and disintegration of complex carbohydrates are largely dependent on the fundamental carbohydrate chemistry reactions of hydrolysis and dehydration. knowing the structure, purpose, and metabolism of carbohydrates depends on knowing these processes, which entail the addition or removal of water molecules, respectively.
When water is added to a chemical reaction called hydrolysis, a molecule is disassembled into smaller parts. In the chemistry of carbohydrates, glycosidic bonds that bind monosaccharides together are frequently broken through hydrolysis processes. The glycosidic link is broken during the process, and one monosaccharide unit is attached to one by the hydroxyl (OH) group from water and the other by the hydrogen (H) group. Two distinct monosaccharide units are created as a result of this. Both inside our bodies and in lab settings for the characterization and study of carbohydrates, hydrolysis reactions are crucial for the digestion of complex carbs.
On the other hand, dehydration reactions entail the removal of water molecules from a reactant, which leads to the formation of a covalent bond. Dehydration reactions are crucial in the formation of glycosidic linkages, which link monosaccharides to generate disaccharides, oligosaccharides, and polysaccharides in the study of carbohydrates. A water molecule is produced as a byproduct of the dehydration synthesis when a hydroxyl (OH) group from one monosaccharide and a hydrogen (H) atom from another combine. The two monosaccharide units join together in a glycosidic bond as a result of this action. The synthesis of the carbohydrates found in plants, animals, and microbes depends on dehydration reactions.
Both hydrolysis and dehydration reactions are reversible processes, which means that depending on the circumstances, they can go either way. In living things, the equilibrium between these processes is carefully managed, enabling the controlled breakdown and synthesis of carbohydrates as required.
Enzymatic Reactions
Because they are responsible for the metabolism and synthesis of carbohydrates in living organisms, enzymatic processes play a significant role in the chemistry of carbohydrates. Specialized proteins called enzymes serve as catalysts for biological reactions, enabling and accelerating them without being eaten. Enzymes play a role in a number of metabolic pathways and help to regulate and manage carbohydrate metabolism in the setting of carbohydrate chemistry.
Numerous processes are catalyzed by the enzymes involved in carbohydrate metabolism. The process of converting glucose into energy, known as glycolysis, is one of the most significant enzyme reactions. In this pathway, a sequence of processes that transform glucose into pyruvate are successively catalyzed by enzymes, producing ATP (adenosine triphosphate) in the process.
Gluconeogenesis, or the production of glucose from non-carbohydrate substrates, also involves enzymes. During fasting or low-carbohydrate diets, the body can maintain blood glucose levels thanks to this mechanism, which largely takes place in the liver and kidney cells. Key roles in gluconeogenesis are played by enzymes like glucose-6-phosphatase, fructose-1,6-bisphosphatase, and phosphoenolpyruvatecarboxykinase.
Enzymes are also necessary for the creation and destruction of complex carbohydrates. For instance, the enzyme glycogen synthase is in charge of accelerating the creation of glycogen, a branching glucose polymer that serves as an animal's main source of glucose storage. On the other hand, enzymes like glycogen phosphorylase and -amylase help break down starch and glycogen, releasing glucose for the creation of energy.
In the study of carbohydrates, enzymatic processes are extremely specialized and strictly controlled. The ability of enzymes to recognize and interact with particular substrates enables them to favor some processes while rejecting others. Allosteric regulation, feedback inhibition, post-translational modifications, and other methods are used to control enzyme activity, ensuring that carbohydrate metabolism is precisely adjusted to satisfy the needs of the organism.
Conclusion
To successfully complete carbohydrate chemistry assignments, it's imperative to understand the fundamental ideas and reactions involved. Students may confidently assess and resolve issues relating to monosaccharides, glycosidic linkages, mutarotation, oxidation, reduction, hydrolysis, dehydration, and enzymatic processes by knowing the structure, characteristics, and reactions of carbohydrates. Students can succeed in carbohydrate chemistry assignments and get a greater understanding of the subject by having a solid foundation in these essential ideas.