Quantum Chemistry Assignment Help: Exploring the Fundamentals
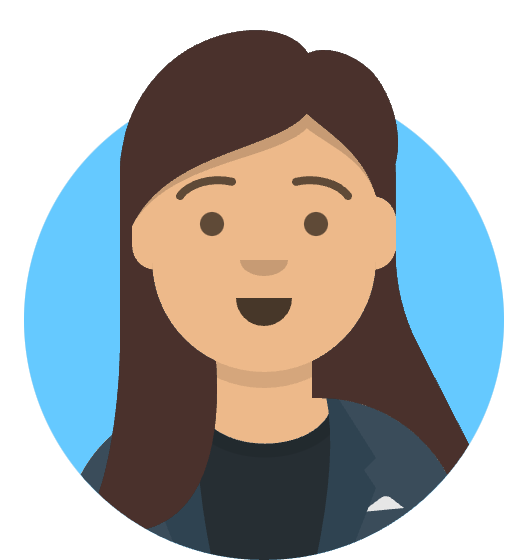
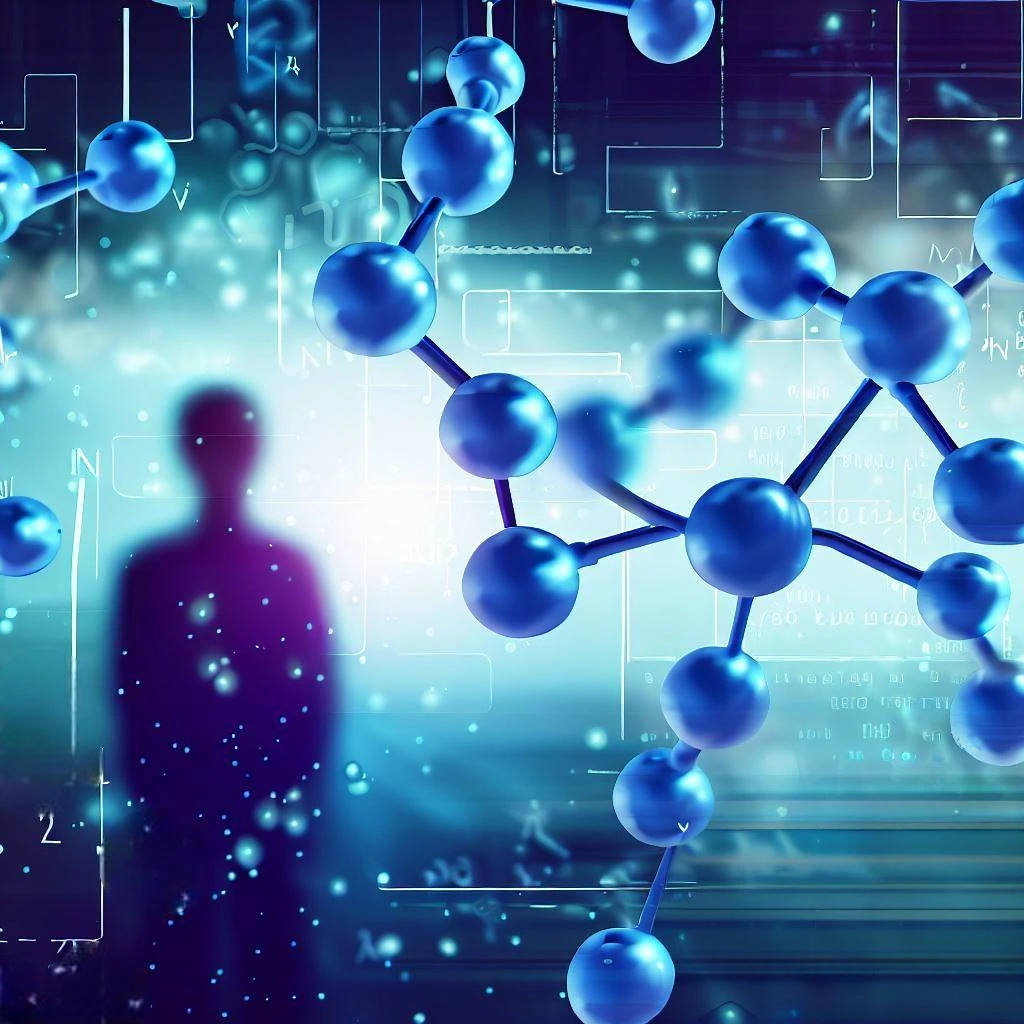
The study of chemical systems at the atomic and molecular levels using the concepts of quantum mechanics is the subject of the fascinating field of chemistry known as quantum chemistry. It offers a deeper comprehension of atoms' and molecules' interactions, behavior, and underlying physical processes. For students studying this subject, the complexities of quantum chemistry can occasionally present difficulties. You're not the only one who struggle to complete quantum chemistry Assignment. In this blog, we'll look at the fundamentals of quantum chemistry and talk about how getting expert assignment help can help you learn this challenging subject.
The Basics of Quantum Chemistry
For the purpose of describing and analyzing the behavior of atoms and molecules, quantum chemistry combines the concepts of quantum mechanics with conventional chemistry methods. At its core, quantum mechanics is concerned with the wave-particle duality of matter, wherein particles like electrons are described as wave functions rather than as classical particles with clearly defined positions because they are more likely to exist in a variety of states.
Understanding Wave Functions and Quantum States
Wave functions are essential for describing the behavior of particles in quantum chemistry. A mathematical function that describes a particle's quantum state is called a wave function and is represented by the Greek letter Psi (). The probability density of finding the particle at a specific position can be found by taking the wave function's square (|||2) into account.
Wave-Particle Duality
The wave-particle duality, which holds that particles can behave both like waves and like particles, is one of the fundamental ideas in quantum mechanics. This implies that in addition to acting as localized particles, particles like electrons can display wave-like characteristics, such as interference and diffraction.
The well-known double-slit experiment serves as the best example of wave-particle duality. An interference pattern is seen on the screen behind the slits in this experiment when a beam of electrons or photons is passed through two closely spaced slits, demonstrating the wave-like nature of particles. However, the particles behave discretely and exhibit particle-like behavior when observed or measured at the slits.
Quantum Superposition
Superposition, which enables particles to exist in multiple states at once, is a key idea in quantum mechanics. Until it is measured or observed, an electron can exist in a superposition of different energy levels or orbital states before collapsing into one of those states.
An electron in an atom, for instance, can coexist in a superposition of various orbitals, each of which corresponds to a different energy level. The electron doesn't "choose" one of the possible states and collapses into that particular state until a measurement is made, such as determining the electron's position or energy.
Quantum Operators and Observables
In quantum systems, the behavior of particles is described by quantum operators, such as the Hamiltonian operator. By applying these operators to the wave function, different observables, including energy, position, and momentum, are obtained. The observables' potential values are represented by the eigenvalues of these operators.
The total energy of a quantum system is represented by the Hamiltonian operator, H. The energy eigenvalues and eigenfunctions, which offer details on the system's energy levels and associated wave functions, can be computed by applying the Hamiltonian operator to the wave function. To calculate observables like position and momentum, respectively, one can use other operators, such as the position operator (x) and the momentum operator (p).
Principles of Quantum Chemistry
Several concepts and models have been developed to integrate the study of chemistry with quantum mechanics. Here are two crucial tenets of quantum chemistry:
The Pauli Exclusion Principle
According to Wolfgang Pauli's formulation of the Pauli exclusion principle, no two electrons in an atom can have the same set of quantum numbers. The elements' electronic structure and periodicity are determined by this principle, which also guarantees the stability of atoms.
Each electron in an atom can be described by a distinct set of quantum numbers, including the principal quantum number (n), azimuthal quantum number (l), magnetic quantum number (m), and spin quantum number (s), in accordance with the Pauli exclusion principle. The electron's energy level, orbital shape, orientation, and spin orientation are all determined by these quantum numbers. According to the principle, two electrons cannot share the same set of quantum numbers and cannot coexist in the same orbital.
Understanding atoms' electronic configuration and predicting their chemical properties require an understanding of the Pauli exclusion principle. The formation of chemical bonds and the stability of matter are explained by the way electrons arrange themselves in various energy levels and orbitals.
The Born-Oppenheimer Approximation
The separation of nuclear and electronic motions is made possible by the Born-Oppenheimer approximation, which bears the names of Max Born and J. Robert Oppenheimer. It works under the premise that the motion of atomic nuclei moves much more slowly than that of electrons, allowing for the treatment of nuclear and electronic motions independently.
The Born-Oppenheimer approximation streamlines the mathematical treatment of molecular systems by separating the nuclear and electronic motions. Without specifically taking into account nuclear motion, it enables the determination of the electronic structure of molecules, including their molecular orbitals and energies. This approximation is based on the notion that because nuclei are much heavier than electrons, changes in the electronic distribution affect them more slowly.
In quantum chemistry, the Born-Oppenheimer approximation is frequently used to examine molecular characteristics like bond lengths, bond angles, and vibrational frequencies. It serves as the foundation for many computational techniques used to analyze and forecast the spectroscopic properties of molecular systems.
Applications of Quantum Chemistry
Applications for quantum chemistry are numerous in many branches of science and technology. The following are three crucial areas where quantum chemistry is crucial:
Molecular Structure and Spectroscopy
The structure, stability, and characteristics of molecules can be determined with the aid of quantum chemistry. Understanding spectroscopic methods like UV-visible spectroscopy, infrared spectroscopy, and nuclear magnetic resonance (NMR) spectroscopy requires knowledge of the electronic structure of molecules, their molecular orbitals, and the energy levels connected to various electronic transitions.
The absorption and emission spectra of molecules, which reveal important details about their electronic and vibrational properties, can be predicted and interpreted by researchers using quantum mechanical principles. This information is essential for comprehending how molecules behave during chemical reactions, identifying unidentified compounds, and creating new materials with particular properties.
Computational Drug Discovery
In computational drug discovery, where scientists use computer simulations to forecast the behavior of molecules in drug design and development, quantum chemistry is crucial. To better understand the binding affinity, selectivity, and reaction mechanisms involved in drug action, researchers can study the interactions between drug molecules and target proteins at the atomic level by using quantum mechanical calculations.
Researchers can screen huge databases of chemical compounds, predict their biological activity, and optimize their properties using computational methods based on quantum chemistry, such as molecular docking, molecular dynamics simulations, and quantum chemical calculations. These techniques help create effective and secure drugs. By limiting the experimental search space and directing the synthesis of new drug candidates, this method conserves time and resources.
Materials Science and Nanotechnology
Due to its ability to shed light on the electronic and optical characteristics of materials at the nanoscale, quantum chemistry is an essential component of materials science and nanotechnology. Quantum mechanical calculations are useful for predicting the electronic band structures, energy levels, and transport characteristics of electrons in nanostructures like quantum dots, nanowires, and graphene.
Researchers can create materials with specialized properties for uses such as electronics, energy storage, and conversion, catalysis, and sensors by studying the quantum effects that occur at the nanoscale. Nanotechnology advancements in fields like quantum computing, photovoltaics, and nanomedicine have been made possible by quantum chemistry's capacity for the design and optimization of nanomaterials with desired properties.
Conclusion
A strong conceptual framework for comprehending the behavior of atoms and molecules at the quantum level is provided by quantum chemistry. You can gain a deeper understanding of the principles governing chemical systems and their applications in various fields by mastering the fundamentals of quantum chemistry. But if you're having trouble with your quantum chemistry assignments, getting professional assistance can give you the direction and encouragement you need to do well in this challenging subject. So embrace quantum chemistry's wonders and set out on a journey of exploration and learning!