Unlocking Molecular Secrets: Organic Spectroscopy Methods for Assignment Success
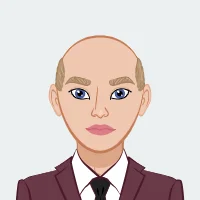
In the realm of organic chemistry, where molecules dance with complexity, unraveling their intricate structures stands as a fundamental challenge. Assignments and research endeavors often demand a profound comprehension of the composition and connectivity of organic compounds. Here, Organic Spectroscopy emerges as an indispensable ally—a set of powerful techniques that play a pivotal role in analyzing molecular structures. This comprehensive guide will embark on a journey into the fascinating world of help with your organic chemistry assignment Organic Spectroscopy, with a particular focus on two key methods: Nuclear Magnetic Resonance (NMR) and Infrared (IR) spectroscopy.
Organic Spectroscopy serves as the lens through which chemists can peer into the molecular landscapes, deciphering the code that dictates their behavior and properties. At the heart of this exploration are NMR and IR spectroscopy, two techniques that have become indispensable tools in the hands of chemists.
Nuclear Magnetic Resonance (NMR) spectroscopy allows scientists to probe the magnetic properties of atomic nuclei within a molecule. By subjecting a sample to a strong magnetic field and radiofrequency radiation, NMR spectroscopy unveils unique signals corresponding to different nuclei in the sample. The positions and intensities of these signals provide crucial information about the molecular environment, aiding in the determination of the compound's structure. NMR has proven to be a versatile and powerful technique, capable of unraveling complex structures and offering insights into the dynamics of molecular interactions.
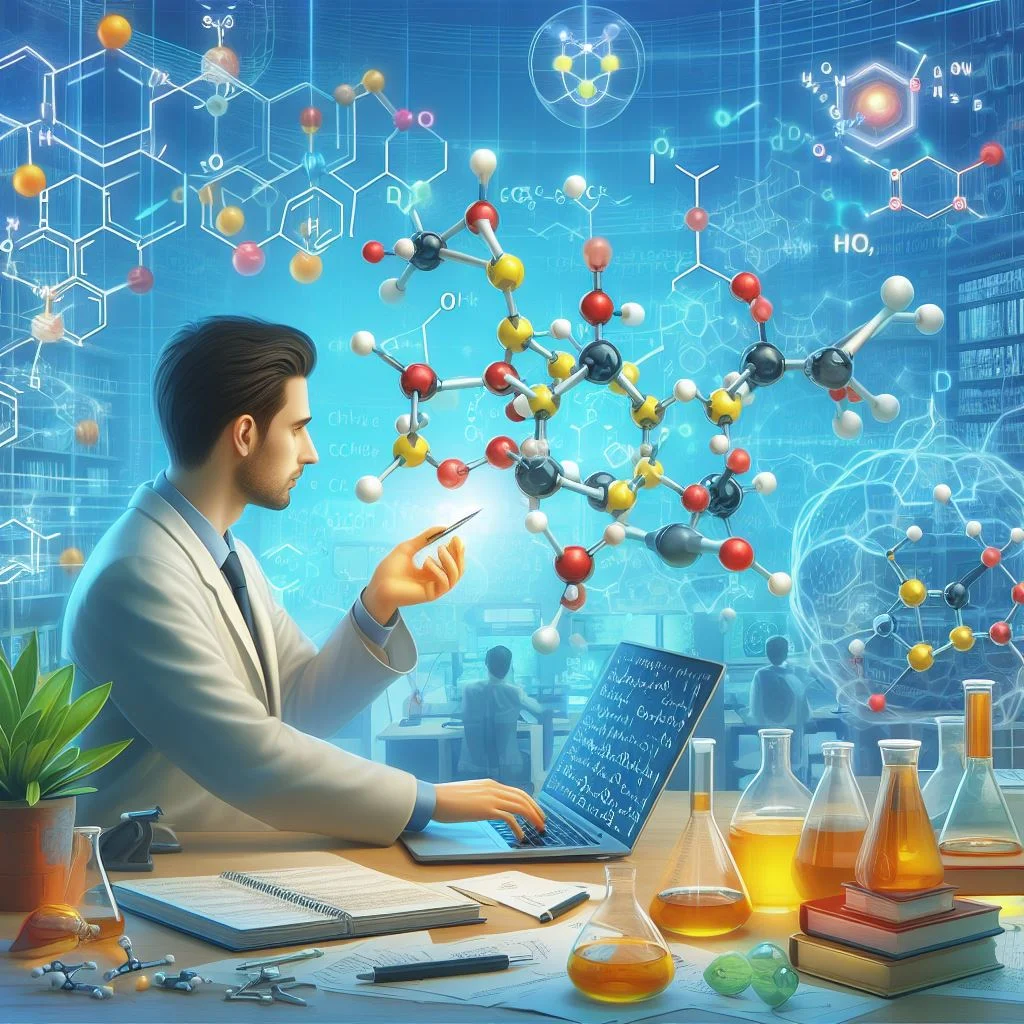
Infrared (IR) spectroscopy, on the other hand, capitalizes on the absorption of infrared radiation by molecular vibrations. Every chemical bond possesses a characteristic vibrational frequency, and when exposed to infrared light, these bonds absorb energy, leading to distinctive peaks in the IR spectrum. This technique provides a fingerprint of a molecule's functional groups, enabling chemists to identify and characterize compounds. IR spectroscopy is particularly valuable in elucidating the presence of key functional groups such as carbonyls, alcohols, and amines.
Navigating through the intricacies of NMR and IR spectroscopy requires a fundamental understanding of the underlying principles. In NMR, the concept of nuclear spin and the interaction of nuclei with an external magnetic field lay the groundwork. Understanding chemical shift, coupling constants, and relaxation processes becomes paramount in interpreting NMR spectra accurately. Meanwhile, IR spectroscopy relies on the resonance of molecular vibrations and the absorption of specific wavelengths, demanding an appreciation for the diverse vibrational modes associated with different functional groups.
Practical applications of these techniques abound across various branches of chemistry. In medicinal chemistry, NMR spectroscopy aids in elucidating the three-dimensional structures of bioactive molecules, guiding the design of pharmaceuticals. In environmental chemistry, IR spectroscopy proves invaluable in analyzing pollutants and identifying contaminants in air and water. The synergy of NMR and IR spectroscopy extends into material science, forensics, and beyond, making them indispensable tools for scientists across disciplines.
As technology advances, so does the sophistication of Organic Spectroscopy. Modern NMR instruments equipped with advanced pulse sequences and multidimensional experiments enhance the resolution and sensitivity of spectra, enabling the characterization of increasingly complex molecules. Infrared spectroscopy benefits from innovations such as Fourier-transform infrared (FT-IR) spectrometers, providing rapid and high-resolution data acquisition.
Understanding Organic Spectroscopy
Organic Spectroscopy stands as a pivotal branch within the realm of chemistry, dedicated to the meticulous exploration of the interaction between organic compounds and electromagnetic radiation. This field employs a diverse array of spectroscopic techniques to unravel the intricacies of molecular structures, compositions, and bonding patterns. Through the interplay of light and matter, researchers gain profound insights into the fundamental nature of organic compounds.
The significance of Spectroscopy extends beyond the confines of laboratory research; it holds a crucial role in academic assignments, offering an indispensable tool for chemistry students and professionals alike. In the intricate world of organic chemistry, accurately identifying and elucidating the structures of compounds is paramount. Spectroscopy emerges as a beacon, providing a non-destructive and highly accurate means to achieve this goal.
One of the key strengths of Spectroscopy lies in its non-destructive nature. Unlike some chemical analysis methods that alter or consume the sample, spectroscopic techniques enable researchers to probe the molecular landscape without compromising the integrity of the compound. This non-invasive quality is particularly valuable when working with limited or precious samples.
Moreover, the accuracy of Spectroscopy adds another layer of significance to its role in assignments. Students tasked with unraveling the mysteries of organic compounds benefit from the precision offered by spectroscopic methods. Whether identifying functional groups, discerning isomeric structures, or deducing the nature of chemical bonds, Spectroscopy equips learners with a powerful and reliable set of tools.
Professionals in the field also rely heavily on Spectroscopy for its ability to provide comprehensive insights into molecular structures. From pharmaceutical research to environmental analysis, the applications are far-reaching. As technology continues to advance, so does the prowess of spectroscopic techniques, ensuring that Organic Spectroscopy remains at the forefront of analytical chemistry, driving discoveries and solving complex challenges in the intricate world of organic compounds.
Unveiling the Power of NMR Spectroscopy
Nuclear Magnetic Resonance (NMR) spectroscopy stands as a cornerstone in the realm of analytical techniques, offering unparalleled insights into the structural intricacies of molecules. At its core, NMR harnesses the magnetic properties exhibited by certain atomic nuclei, providing a non-destructive means of unraveling the mysteries of molecular architecture.
The fundamental principle underlying NMR involves subjecting a sample to a strong magnetic field and subsequently bombarding it with radiofrequency radiation. The response of atomic nuclei to these stimuli yields a spectrum of signals, each unique to the specific nuclei in the sample. This spectrum, often represented graphically, serves as a molecular fingerprint, allowing researchers to decipher valuable information about the local environment of atoms within the molecule.
One of the key strengths of NMR spectroscopy lies in its ability to elucidate the connectivity of atoms in a molecule. By meticulously analyzing the signals emitted by atomic nuclei, researchers can deduce not only the types of atoms present but also their spatial relationships. This capability is particularly invaluable in determining the three-dimensional arrangement of atoms, providing a level of detail that other analytical techniques may struggle to achieve.
In the realm of assignments, NMR spectroscopy emerges as a powerful tool for structural analysis. Whether tackling the elucidation of a complex natural product or verifying the success of a synthetic reaction, NMR serves as a game-changer. Real-world applications abound, with researchers relying on NMR data to confirm molecular structures, identify functional groups, and discern stereochemistry.
Consider a scenario where a chemist embarks on the synthesis of a novel compound. NMR spectroscopy becomes instrumental in confirming the desired product's formation, revealing the presence of specific functional groups and verifying the absence of unwanted by-products. The ability to precisely analyze the spectral data allows researchers to troubleshoot synthetic pathways, ensuring the reproducibility and reliability of their experiments.
Furthermore, in natural product chemistry, where the molecular structures can be highly intricate, NMR spectroscopy plays a pivotal role in characterizing these compounds. Researchers can unravel the complex web of atoms and bonds, piecing together the puzzle of a compound's structure with confidence.
Peering into Molecular Vibrations with IR Spectroscopy
Infrared (IR) spectroscopy is a powerful analytical technique that delves into the fascinating realm of molecular vibrations induced by the absorption of infrared radiation. This method plays a pivotal role in elucidating the intricate details of a molecule's structure by providing insights into the functional groups it harbors.
At its core, IR spectroscopy hinges on the interaction between infrared radiation and molecular vibrations. When infrared light is absorbed by a molecule, it imparts energy to specific bonds, causing them to vibrate. The resulting vibrational frequencies are characteristic of different functional groups, offering a unique fingerprint for the molecular composition. This makes IR spectroscopy an invaluable tool for chemists and researchers aiming to unravel the complex architecture of organic compounds.
One of the primary strengths of IR spectroscopy lies in its ability to pinpoint functional groups within a molecule. Functional groups are specific arrangements of atoms that confer distinct chemical properties to a compound. From carbonyl groups to hydroxyl groups, IR spectroscopy enables a detailed analysis of these key components. By identifying and understanding the presence of these functional groups, scientists can glean essential information about a molecule's behavior and reactivity.
In the realm of structural assignments, IR spectroscopy serves as a complementary partner to Nuclear Magnetic Resonance (NMR). While NMR provides information about the connectivity of atoms in a molecule, IR spectroscopy offers crucial details about the functional groups present. This synergy enhances the accuracy of structural assignments by cross-validating information obtained from both techniques.
The marriage of NMR and IR spectroscopy is particularly potent in confirming structural details and elucidating ambiguous cases. For example, if NMR data suggests the presence of a certain functional group but leaves room for uncertainty, IR spectroscopy can step in to either affirm or negate the initial hypothesis. This collaborative approach enhances the reliability of structural assignments, reducing the margin for error in chemical analyses.
Practical examples abound to illustrate the symbiotic relationship between NMR and IR spectroscopy. Researchers routinely encounter complex organic molecules with ambiguous structural features. By integrating data from both techniques, they can construct a more comprehensive and accurate picture of the molecule under investigation. This holistic approach contributes significantly to advancing our understanding of molecular structures and facilitates breakthroughs in diverse scientific fields, from drug discovery to materials science.
Integrating NMR and IR for Comprehensive Structural Analysis
Nuclear Magnetic Resonance (NMR) and Infrared (IR) spectroscopy are powerful analytical techniques that provide unique insights into the structure of molecules. However, their true potential is realized when these two methods are combined, creating a synergistic approach that enhances the accuracy and depth of structural analysis.
This integration is pivotal in unraveling complex molecular structures, and a step-by-step guide will be provided to empower readers in effectively merging NMR and IR data in assignments. The process involves interpreting NMR spectra alongside correlating IR peaks with specific functional groups, offering a comprehensive understanding of the molecular composition.
The guide aims to equip students and researchers with the skills needed to tackle challenging structural elucidation tasks. Understanding the intricacies of both NMR and IR spectra allows for a more holistic analysis, enabling researchers to overcome hurdles that might be insurmountable when using each technique in isolation.
The content delves into real-world case studies that serve as exemplars, showcasing how the integration of NMR and IR spectroscopy unravels intricate molecular puzzles. These case studies span a spectrum of organic compounds, ranging from natural products to synthetic intermediates. By presenting practical examples, the material provides valuable insights into the application of integrated spectroscopic techniques, facilitating a deeper comprehension of the subject matter.
Overcoming Common Challenges
Spectroscopic analysis, a powerful tool in scientific research, is not without its share of challenges. In this exploration, we delve into the common pitfalls faced by researchers in this field, shedding light on issues such as spectral overlap and ambiguity. Navigating through these obstacles requires a nuanced understanding and strategic approach, and this section aims to empower readers with the knowledge to overcome these challenges in their assignments.
One significant hurdle in spectroscopic analysis is spectral overlap. This phenomenon occurs when two or more substances in a sample produce similar or identical spectra, making it challenging to differentiate and identify individual components accurately. Researchers must grapple with the complexities of distinguishing signals in such overlapping regions, requiring advanced analytical techniques and precise methodologies. Addressing spectral overlap often involves utilizing sophisticated algorithms and deconvolution methods, emphasizing the importance of computational tools in modern spectroscopic analysis.
Ambiguity further complicates the landscape of spectroscopic analysis. Unclear or incomplete data, instrument noise, or unexpected interactions between sample components can introduce ambiguity into the results, hindering the reliability and accuracy of the analysis. Researchers must adopt a systematic approach to address ambiguities, involving thorough validation of experimental conditions, careful calibration of instruments, and meticulous interpretation of results. Additionally, incorporating complementary techniques, such as chromatography or mass spectrometry, can enhance the reliability of spectroscopic findings by providing corroborating information.
To navigate these pitfalls successfully, researchers can employ various strategies. Collaborative efforts between spectroscopists and experts from other disciplines can bring diverse perspectives and innovative solutions. Moreover, continuous education and staying abreast of technological advancements play a crucial role in overcoming challenges in this rapidly evolving field. By acknowledging and proactively addressing pitfalls in spectroscopic analysis, researchers can enhance the robustness of their findings and contribute to the advancement of scientific knowledge in diverse domains. This section serves as a guide, equipping readers with the insights needed to traverse the intricate terrain of spectroscopic analysis effectively.
Conclusion:
Organic Spectroscopy, with its arsenal of techniques such as Nuclear Magnetic Resonance (NMR) and Infrared (IR) spectroscopy, stands as an indispensable tool in the realm of structural analysis for organic compounds. These techniques offer a window into the molecular intricacies that define organic chemistry, providing researchers, scientists, and students with invaluable insights.
NMR spectroscopy, a cornerstone in the field, employs the principles of nuclear magnetic resonance to elucidate the structure of organic compounds. By subjecting a sample to a strong magnetic field and radiofrequency radiation, NMR spectroscopy detects the absorption of energy, unveiling the specific environment of different atomic nuclei. This method allows researchers to discern the connectivity of atoms within a molecule, providing a detailed blueprint of its structure. Through the careful interpretation of NMR spectra, scientists can deduce the arrangement of atoms, the types of bonds present, and even gain information about molecular dynamics.
In parallel, IR spectroscopy harnesses the interaction between molecules and infrared radiation. As molecules absorb specific frequencies of infrared light, their vibrational modes become apparent in the resulting spectrum. This technique is particularly adept at revealing functional groups within a molecule, enabling the identification of key features that contribute to its overall structure. IR spectroscopy complements NMR by offering a different perspective on molecular composition, enhancing the depth of structural analysis.
The integration of NMR and IR techniques transforms organic chemistry assignments from routine tasks into opportunities for exploration and discovery. Understanding the principles behind these methods is akin to wielding a powerful set of analytical tools, allowing students to unravel the mysteries locked within the molecular structures they encounter. Assignments become more than mere exercises; they become gateways to a deeper comprehension of the complexities inherent in organic compounds.
For students, mastering Organic Spectroscopy opens doors to enhanced assignment performance. The ability to apply these techniques not only demonstrates proficiency but also fosters a profound appreciation for the elegance of molecular design. It is a journey into the microscopic world, where seemingly complex structures are unveiled with precision and clarity.
In conclusion, Organic Spectroscopy, with a focus on NMR and IR techniques, empowers individuals engaged in the study of organic compounds. The symbiosis between theory and practice, principles and applications, elevates the learning experience. Embarking on this journey allows students to transcend the confines of routine assignments, transforming them into avenues for intellectual exploration and scientific discovery. The world of Organic Spectroscopy beckons, promising a rich landscape of knowledge and understanding for those willing to delve into its depths.