Ligand Design in Coordination Chemistry: Strategies for Complex Assignments
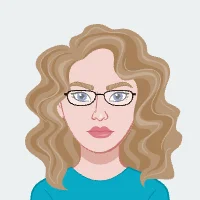
Coordination chemistry stands as a captivating domain within the expansive realm of inorganic chemistry. Its allure lies in the intricate choreography of metal ions and ligands within coordination compounds, offering a rich tapestry of applications across catalysis, medicine, and materials science. In this exploration of coordination chemistry, this blog post endeavors to unravel the nuanced strategies and considerations that underlie ligand design, particularly when faced with complex chemistry assignments.
At its core, coordination chemistry focuses on the interactions between metal ions and ligands, molecular species that donate electrons to the metal center. These coordination compounds exhibit a diversity of structures and properties, forming the basis for a plethora of practical applications. Catalysis, for instance, harnesses the unique reactivity of coordination compounds to facilitate chemical transformations. The coordination of metal ions with ligands can significantly enhance the efficiency and selectivity of catalytic processes, playing a pivotal role in industrial and environmental applications.
Medicine benefits from coordination chemistry through the design of metal-based drugs. Transition metal complexes, often involving carefully selected ligands, exhibit biological activity that can be tailored for therapeutic purposes. Coordination compounds have been explored for their potential in anticancer agents, imaging agents, and treatments for various diseases. The precise control over ligand design becomes crucial in modulating the biological activity and toxicity of these metal-based drugs.
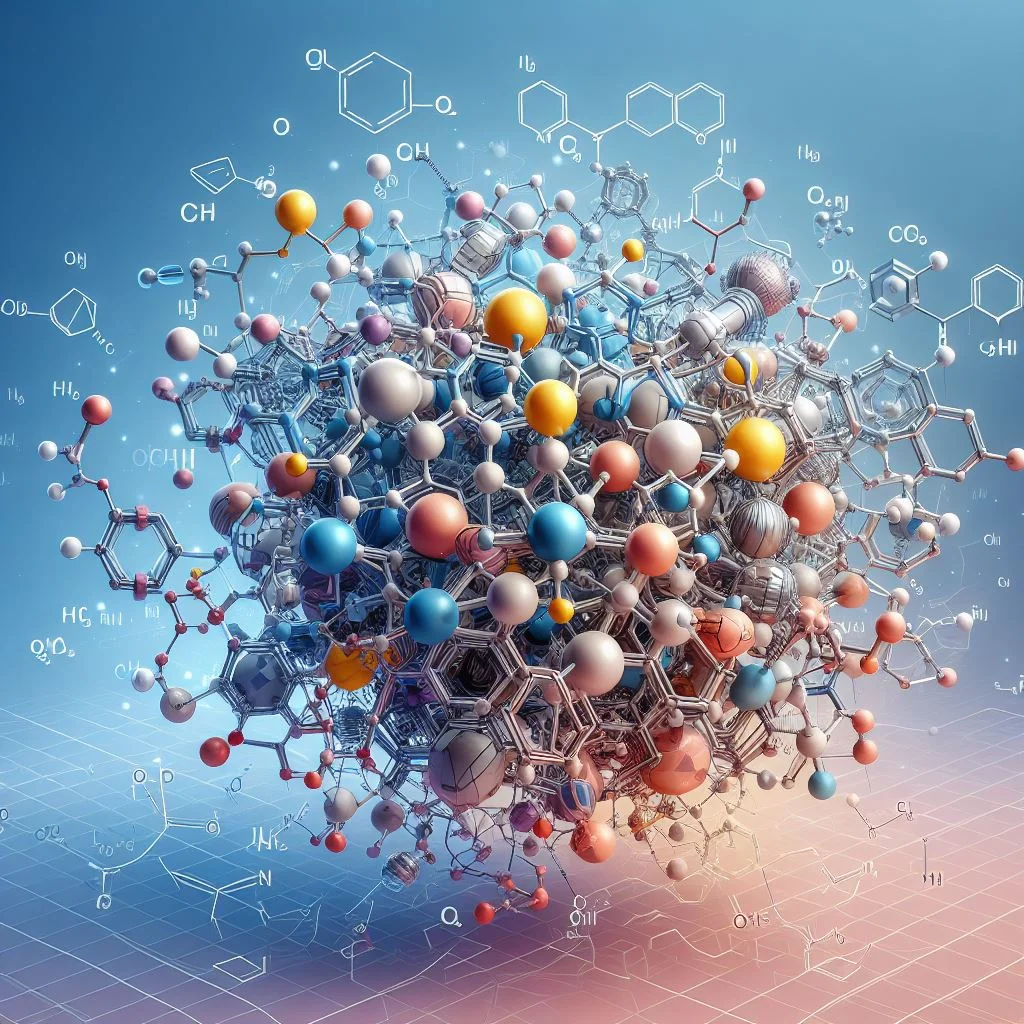
Materials science also reaps the rewards of coordination chemistry, where the design of ligands influences the properties of coordination polymers and metal-organic frameworks (MOFs). These materials find applications in gas storage, separation, and sensing. Ligands play a pivotal role in determining the porosity, stability, and selectivity of these materials, showcasing the significance of thoughtful ligand design in achieving desired functionalities.
As we delve deeper into the heart of coordination chemistry, the intricacies of ligand design become apparent, especially in the face of complex assignments. Ligands must be tailored to the specific needs of the metal center and the desired properties of the resulting coordination compound. Factors such as sterics, electronics, and chelate effects come into play, influencing the stability and reactivity of the complex. The challenge lies in striking a delicate balance to achieve the desired outcomes.
Understanding Coordination Chemistry
Coordination compounds, integral in chemistry, emerge through the intricate interplay between metal ions and ligands. Ligands, be they molecules or ions, engage with metal ions, giving rise to complex structures with distinctive properties. These coordination complexes play pivotal roles in diverse chemical processes, owing to their unique reactivities. The behavior of coordination compounds is intricately governed by key factors such as coordination number, geometry, and the nature of metal-ligand bonds.
The coordination number denotes the count of ligands attached to the central metal ion, influencing the complex's stability and reactivity. Geometry dictates the spatial arrangement of these ligands around the metal ion, impacting the compound's overall structure. Meanwhile, the nature of metal-ligand bonds, whether they are ionic or covalent, profoundly influences the complex's chemical behavior.
In essence, coordination compounds serve as essential participants in catalysis, medicine, and materials science due to their tailored properties. Their versatility arises from the ability to fine-tune their characteristics by manipulating the coordination number, geometry, and metal-ligand bonds. As a result, understanding these fundamental aspects becomes crucial for unraveling the intricate chemistry behind coordination compounds and harnessing their potential in various applications.
The Significance of Ligand Design
The electronic structure of ligands plays a pivotal role in shaping the behavior of coordination complexes. Chemists strategically modify these properties to fine-tune the redox potential, electron density, and charge distribution within the metal-ligand system. This becomes particularly crucial in reactions involving electron transfer processes. By manipulating the electronic features of ligands, researchers can control the overall reactivity of the metal-ligand complex. For example, tuning the electronic properties facilitates the optimization of catalytic processes and influences the kinetics of reactions involving electron transfer, providing a powerful tool for tailoring the behavior of coordination systems in various applications.
Steric hindrance, governed by the spatial arrangement of atoms within a ligand, profoundly impacts ligand design. Bulky ligands influence the accessibility of the metal center, thereby affecting the coordination number and geometry. Chemists carefully consider steric effects in scenarios where spatial constraints can dictate the outcome of a reaction. This involves balancing the desire for stability with the need for reactivity. Understanding steric considerations is crucial for achieving the desired outcome in metal-ligand interactions, especially in the development of catalysts and the design of coordination complexes for specific applications.
Chelating ligands, characterized by multiple binding sites, play a significant role in the design of coordination complexes. These ligands form stable rings or cages with metal ions, a phenomenon known as the chelate effect. The increased stability provided by chelating ligands is particularly valuable in complex assignments where enhanced stability is desired. Ethylenediamine and 2,2'-bipyridine are examples of chelating ligands commonly employed in coordination chemistry. The chelate effect not only improves the stability of the coordination complex but also influences the selectivity of metal binding, making these ligands versatile tools in the design of molecular systems with specific functions.
Incorporating diverse functional groups into ligand structures expands the range of interactions with metal ions. This diversity leads to the formation of unique coordination environments, allowing for a tailored approach to complex assignments. Common functional groups, such as amine, carbonyl, and phosphine, offer different binding modes and contribute to the versatility of ligands. The presence of varied functional groups influences the overall reactivity of the coordination complex, impacting its suitability for specific applications. By strategically incorporating different functional groups, chemists can design ligands with specific properties, enabling the customization of coordination complexes for targeted purposes in catalysis, materials science, and other fields of research.
Strategies for Ligand Synthesis
The synthesis of ligands stands as a pivotal step in the intricate process of designing coordination complexes, and researchers have developed several strategies to streamline this synthesis, ensuring efficiency and reproducibility. These strategies play a crucial role in addressing the challenges posed by complex assignments in coordination chemistry.
Organometallic precursors, characterized by metal-carbon bonds, represent a significant avenue for ligand synthesis. These precursors serve as starting materials, offering a unique advantage by imparting enhanced compatibility with specific metal ions. Ligands derived from organometallic precursors can be tailored to exhibit specific characteristics, allowing for a more nuanced and customized approach to coordination chemistry. This strategy aligns with the idea that the choice of ligand profoundly influences the properties and reactivity of coordination complexes.
Template synthesis introduces a different approach, utilizing pre-existing structures known as templates to guide the assembly of ligands around a central metal atom. This method affords precise control over ligand geometry, enabling the creation of complex structures with well-defined coordination environments. By leveraging template synthesis, researchers can engineer coordination complexes with desired properties, optimizing their functionality for various applications.
Click chemistry approaches have also found their place in ligand synthesis. Click chemistry, known for its emphasis on high-yield and modular reactions, provides a valuable tool for rapidly generating diverse ligands. The simplicity and efficiency of click chemistry make it an attractive option for researchers seeking to address complex assignments. This approach contributes to the versatility of ligand synthesis, offering a platform that can be adapted to different coordination scenarios.
Combinatorial chemistry techniques take a more high-throughput approach, enabling the simultaneous synthesis of a large number of ligands with slight structural variations. This approach accelerates the exploration of ligand libraries, facilitating the identification of optimal ligands for specific coordination scenarios. The ability to rapidly generate and assess a variety of ligands is particularly advantageous when facing intricate coordination challenges, allowing researchers to efficiently navigate the vast landscape of possible ligand structures.
Metal-Ligand Interactions: A Dynamic Perspective
Understanding the dynamic nature of metal-ligand interactions is paramount in successfully navigating complex assignments in coordination chemistry. The interplay between metal ions and ligands is not static; it involves a delicate equilibrium influenced by various factors such as temperature, solvent, and concentration.
Coordination dynamics, the first aspect discussed, sheds light on the dynamic changes occurring in the coordination sphere around a metal ion. This dynamism is a result of various processes, including ligand exchange reactions, isomerization, and redox processes. Ligand exchange reactions involve the replacement of one or more ligands in the coordination sphere, altering the structure and properties of the complex. Isomerization refers to the rearrangement of atoms within the coordination sphere, leading to different isomeric forms of the complex. Redox processes involve changes in the oxidation states of the metal ion, contributing to the overall flexibility and adaptability of coordination complexes. Recognizing and manipulating these dynamics are key to achieving desired outcomes in complex assignments, allowing chemists to tailor coordination complexes for specific applications.
Solvent effects, the second consideration, highlight the significant impact of the choice of solvent on the stability and reactivity of coordination complexes. Solvent molecules can coordinate to the metal center, altering the coordination environment and influencing the overall behavior of the complex. This solvent-induced coordination can lead to changes in the geometry, electronic structure, and reactivity of the metal-ligand system. Chemists must carefully consider solvent effects when designing ligands for specific applications, as the solvent environment can profoundly influence the performance and properties of coordination complexes.
Temperature-dependent behavior, the third aspect, emphasizes the crucial role of temperature in determining the thermodynamic and kinetic aspects of metal-ligand interactions. Changes in temperature can affect the equilibrium constants of coordination reactions, influencing the formation of different coordination species. Ligand design strategies must account for the temperature dependence of coordination processes to ensure the reproducibility and stability of the desired complex under varying conditions. Temperature serves as a tunable parameter, allowing chemists to control the outcome of coordination reactions and tailor the properties of coordination complexes based on specific temperature requirements.
Case Studies: Applying Ligand Design to Real-World Challenges
Ligand design stands at the forefront of scientific innovation, offering versatile strategies that find practical applications across various domains. In the realm of catalysis for green chemistry, ligand design emerges as a key player in the quest for sustainable solutions. Through meticulous tailoring of ligands with specific electronic and steric properties, researchers have successfully developed catalysts that not only drive chemical reactions but also do so with reduced environmental impact. These tailored ligands enable the synthesis of valuable chemicals, contributing to the evolution of green and sustainable chemistry practices.
In the domain of medicinal coordination complexes, ligand design takes center stage in the pursuit of therapeutic breakthroughs. The precision control of ligand structures has proven instrumental in the creation of coordination complexes with enhanced therapeutic properties. This has paved the way for the development of metal-based drugs that exhibit heightened efficacy while minimizing undesirable side effects. The ability to fine-tune ligand structures opens new frontiers in medicinal chemistry, offering researchers a powerful tool to design drugs with unprecedented precision and effectiveness.
Materials science, another arena where ligand design showcases its prowess, witnesses the creation of cutting-edge materials with tailored properties. From luminescent materials used in advanced display technologies to conductive materials crucial for electronic applications, ligand design strategies provide the means to finely adjust material characteristics to meet specific requirements. The versatility of ligand design in materials science contributes to the development of innovative solutions that drive advancements in various industries, from electronics to energy.
These case studies underscore the transformative impact of ligand design strategies in addressing complex challenges and advancing scientific frontiers. The synergy between ligand design and catalysis not only promotes green chemistry but also aligns with global efforts towards sustainability. In medicinal chemistry, the precision afforded by ligand design holds promise for the development of safer and more effective drugs. Meanwhile, materials science benefits from the adaptability of ligand design, fostering the creation of materials tailored to diverse applications.
Future Directions and Emerging Trends
As our understanding of coordination chemistry advances, the field presents intriguing challenges and promising opportunities. Delving into the frontiers of ligand design, researchers are exploring novel approaches that have the potential to revolutionize catalysis and medicinal chemistry. Three particularly captivating avenues in this exploration are bio-inspired ligands, smart ligand systems, and computational ligand design.
Bio-inspired ligands, the first frontier, draw inspiration from the efficiency and selectivity observed in biological systems. By mimicking the intricate designs found in nature, scientists aim to enhance the performance of ligands in various applications. These bio-inspired ligands hold the promise of unlocking new avenues for catalysis and medicinal chemistry. Learning from the exquisite coordination of metals in biological molecules, researchers strive to create ligands that exhibit similar precision and efficacy, bringing a biomimetic touch to synthetic chemistry.
The second frontier, smart ligand systems, introduces a paradigm shift in ligand design. This emerging area of research involves integrating responsive elements into ligand structures, creating intelligent systems that can adapt their conformation or properties in response to external stimuli. The ability to control metal-ligand interactions with precision opens up possibilities for dynamic and tunable catalytic processes. These ligands could respond to specific environmental conditions or trigger events, providing unprecedented control and flexibility in chemical reactions. The concept of ligands with a built-in responsiveness represents a new era in tailoring ligand behavior to match the demands of complex chemical processes.
Advancements in computational chemistry form the third frontier, paving the way for the rational design of ligands with desired properties. Virtual screening and molecular modeling techniques play a pivotal role in this process, offering valuable insights into the potential efficacy of ligands. Computational approaches accelerate the ligand design process, allowing researchers to explore a vast design space and prioritize the most promising candidates for synthesis and testing. This synergy between experimental and computational methods marks a significant leap forward in the efficiency and precision of ligand design.
Conclusion
Ligand design in coordination chemistry stands as a vibrant and evolving discipline, continually shaping our comprehension of metal-ligand interactions. This blog post illuminates strategies that empower researchers and chemists to confidently navigate intricate assignments, heralding a new era of possibilities in catalysis, medicine, and materials science. The mastery of these strategies is pivotal, offering a key to unlocking the full potential of metal-ligand systems. The intricate dance between metal ions and ligands is at the forefront of coordination chemistry, revealing a captivating world of molecular interactions.
As we peer into the future, the exploration of ligand design holds the promise of unraveling further secrets within this nuanced field. This ongoing journey is not merely a pursuit of academic curiosity but a gateway to transformative applications. The implications extend to catalytic processes, where tailored ligands can enhance reaction efficiency, pharmaceuticals, where precise metal-ligand interactions may yield targeted therapeutic agents, and materials science, where the properties of materials can be finely tuned for specific applications. In this dynamic landscape, the evolution of ligand design remains integral to advancing our understanding and harnessing the potential of coordination chemistry, ensuring that its impact resonates across diverse scientific domains.