Inorganic Nanomaterials: Synthesis, Properties, and Applications
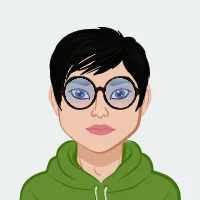
In the ever-evolving landscape of nanotechnology, inorganic nanomaterials have emerged as remarkable entities, showcasing unparalleled versatility and unique properties that have revolutionized various fields. This blog embarks on a fascinating journey through the synthesis methodologies, distinctive properties, and myriad applications that make these materials indispensable. If you need help with your inorganic assignment, delving into the synthesis methodologies and properties of inorganic nanomaterials provides a comprehensive understanding of their significance and applications across diverse fields, offering insights into their transformative potential in various scientific and technological endeavors.
The synthesis of inorganic nanomaterials is a dynamic and intricate process, often tailored to achieve specific properties. Techniques such as sol-gel synthesis, chemical vapor deposition, and hydrothermal methods enable precise control over the size, shape, and composition of these materials. This meticulous manipulation at the nanoscale imparts them with extraordinary characteristics, setting them apart from their bulk counterparts.
One of the defining features of inorganic nanomaterials is their size, typically ranging from 1 to 100 nanometers. This size regime leads to quantum effects and altered physical and chemical properties. Quantum dots, for instance, exhibit size-dependent optical and electronic properties, making them invaluable in applications such as imaging, sensing, and even in the development of quantum computers.
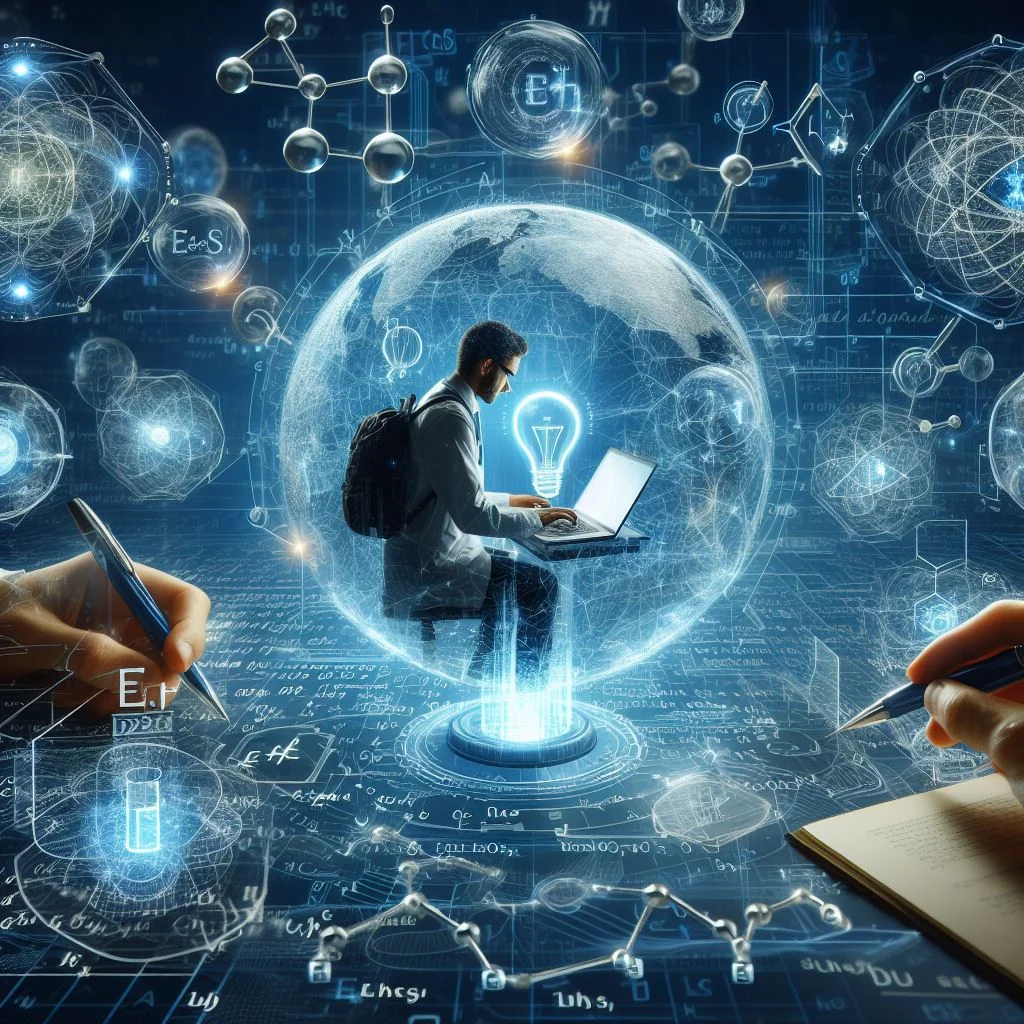
The distinctive properties of inorganic nanomaterials extend beyond size, encompassing aspects like high surface area, enhanced reactivity, and exceptional mechanical strength. These attributes pave the way for a myriad of applications across diverse fields. In medicine, for instance, nanomaterials are employed in drug delivery systems, capitalizing on their ability to target specific cells and tissues with precision. Additionally, the antimicrobial properties of certain nanomaterials contribute to advancements in healthcare by combating drug-resistant bacteria.
In the realm of energy, inorganic nanomaterials play a pivotal role. Nanomaterials like graphene and quantum dots find applications in solar cells, energy storage devices, and catalysis. Their high conductivity, large surface area, and unique electronic properties enhance the efficiency of energy conversion and storage systems, contributing to the development of sustainable technologies.
Furthermore, inorganic nanomaterials have found their way into electronics, catalysis, and environmental remediation. Their versatility enables innovations in fields as diverse as electronics, where nanomaterials are used in the development of high-performance transistors and sensors, and catalysis, where they act as efficient catalysts due to their increased surface area and reactivity.
Understanding Inorganic Nanomaterials
Inorganic nanomaterials represent a fascinating realm of materials science, distinguished by their unique physical and chemical properties arising from their nanoscale dimensions, typically ranging from 1 to 100 nanometers. At this scale, materials exhibit novel behaviors and characteristics, making them integral to various scientific and technological advancements.
Understanding the nature of inorganic nanomaterials is fundamental to appreciating their significance. Their diminutive size and distinctive structures contribute to properties that differ significantly from their bulk counterparts. These materials often showcase enhanced mechanical, electrical, magnetic, and optical attributes, opening up a plethora of possibilities for diverse applications.
The world of inorganic nanomaterials is expansive, encompassing various types that play crucial roles in different fields. Nanoparticles, for instance, are minute particles with dimensions in the nanoscale range. Their high surface area-to-volume ratio makes them particularly interesting for catalysis, drug delivery, and sensing applications. Nanotubes, cylindrical structures with diameters on the nanoscale, exhibit exceptional strength and conductivity, rendering them valuable in fields like materials science and electronics.
Nanowires, elongated structures with diameters in the nanometer range, find applications in electronics, photonics, and sensors due to their unique electronic properties. Quantum dots, semiconductor nanoparticles, exhibit quantum mechanical effects, allowing precise control over their electronic and optical properties. This makes them versatile in applications such as imaging, solar cells, and light-emitting diodes.
The synthesis and manipulation of these inorganic nanomaterials have become focal points of research. Various methods, including chemical vapor deposition, sol-gel processes, and template-assisted approaches, enable the controlled production of nanomaterials with specific properties.
As technology advances, the applications of inorganic nanomaterials continue to expand. From medicine and energy to electronics and environmental remediation, these materials hold immense promise for addressing complex challenges and pushing the boundaries of what is possible at the nanoscale. In the ever-evolving landscape of nanotechnology, exploring the diverse world of inorganic nanomaterials opens doors to innovation and transformative discoveries.
Synthesis Methods
Nanomaterial synthesis is a multidimensional field where researchers employ various strategies to engineer materials at the nanoscale, unlocking unique properties and applications. The synthesis methods can broadly be classified into bottom-up, top-down, and hybrid approaches, each offering distinctive advantages in tailoring the structure and composition of nanomaterials.
Bottom-Up Approaches: One category of synthesis methods involves building nanomaterials from the ground up. The sol-gel process is a prominent example, where a solution undergoes a transformation into a gel and subsequently solid material. This method enables meticulous control over the nanomaterial's structure and composition, crucial for achieving desired properties. Chemical Vapor Deposition (CVD) is another bottom-up technique, where thin films or coatings are formed through the chemical reaction of gaseous precursors. Hydrothermal synthesis, utilizing high-temperature and high-pressure water, is a method particularly useful for creating nanomaterials with specific properties. The bottom-up approaches excel in providing precision and control over the final product.
Top-Down Approaches: Contrastingly, top-down approaches involve manipulating bulk materials to create nanoscale structures. Ball milling is a mechanical technique that breaks down larger materials into smaller particles, contributing to the scalability of nanomaterial production. Lithography, a widely used method in the semiconductor industry, allows for pattern transfer from a mask to a substrate, defining nanoscale features. Laser ablation employs laser beams to remove material, generating nanoparticles. These top-down methods are crucial for their ability to scale up production while maintaining control over the nanoscale characteristics.
Hybrid Approaches: Recognizing the strengths of both bottom-up and top-down methods, hybrid approaches emerge as a balanced strategy. These methods integrate the precision of bottom-up techniques with the scalability of top-down methods, providing a versatile platform for tailoring the properties of inorganic nanomaterials. By combining these approaches, researchers can achieve a synergistic effect, optimizing the benefits of each method to create nanomaterials with enhanced and finely tuned characteristics.
Properties of Inorganic Nanomaterials
In the realm of nanotechnology, inorganic nanomaterials have emerged as a fascinating area of study, showcasing distinctive properties that stem from their diminutive size. A fundamental facet of these materials lies in their size-dependent properties, where phenomena like quantum confinement and surface effects take center stage. As the dimensions of materials shrink to the nanoscale, quantum effects become increasingly pronounced, leading to unique behaviors that differ from those observed in bulk materials. Additionally, surface effects gain prominence, influencing reactivity and interactions at the nanoscale. This interplay of quantum confinement and surface effects is pivotal in conferring inorganic nanomaterials with unparalleled characteristics, making them a subject of intense scientific exploration.
Delving further, the optical properties of inorganic nanomaterials add another layer of complexity and utility. Plasmonic effects and fluorescence, inherent to these materials, open up exciting avenues for practical applications. Plasmonic effects, arising from the collective oscillations of electrons, can be harnessed for enhanced light-matter interactions, paving the way for advancements in sensors, imaging technologies, and optoelectronics. Simultaneously, the fluorescence exhibited by certain nanomaterials holds promise for diverse applications, including bioimaging and sensing.
Beyond the optical realm, the mechanical and electronic prowess of inorganic nanomaterials showcases their transformative potential. These materials exhibit remarkable mechanical strength and electronic properties, propelling them into the forefront of advanced materials and nanoelectronics. The marriage of size-dependent phenomena with exceptional mechanical and electronic attributes positions inorganic nanomaterials as key players in the development of cutting-edge technologies and materials.
Applications of Inorganic Nanomaterials
In the ever-evolving landscape of science and technology, inorganic nanomaterials have emerged as transformative agents across diverse fields, particularly in medicine and healthcare. This microscopic world of materials has revolutionized drug delivery, imaging, and diagnostics, ushering in an era of unprecedented precision. Nanomaterials enable targeted drug delivery, ensuring medications reach specific cells or tissues with enhanced efficiency, minimizing side effects and maximizing therapeutic impact. In imaging and diagnostics, these materials offer unparalleled resolution, allowing for early and accurate disease detection.
Beyond healthcare, the impact of inorganic nanomaterials extends into the realms of energy and the environment. In the pursuit of sustainable technologies, nanomaterials play a pivotal role in energy storage and conversion. Their unique properties enhance the efficiency of batteries and fuel cells, contributing to the development of cleaner and more efficient energy systems. Additionally, these materials prove instrumental in environmental remediation, addressing pollution and fostering cleaner ecosystems.
Electronics and optoelectronics represent another frontier where inorganic nanomaterials showcase their prowess. From improving the performance of electronic devices to enabling the creation of futuristic optoelectronic technologies, these materials drive innovation in the electronics industry. The miniaturization and enhanced functionalities offered by nanomaterials have paved the way for the development of smaller, faster, and more powerful electronic components.
Catalysis and sensing are key domains where nanomaterials demonstrate their versatility. In industrial processes, these materials serve as catalysts, accelerating reactions and improving overall efficiency. Simultaneously, their sensing capabilities make them indispensable in pollution monitoring and chemical detection, contributing to environmental protection and safety.
In essence, inorganic nanomaterials are catalysts of progress across medicine, energy, electronics, and industrial applications. Their microscopic dimensions belie their monumental impact, promising a future where precision, efficiency, and sustainability define technological advancements. As we delve deeper into the nano-scale, the potential for innovation and positive change in our world becomes increasingly limitless.
Challenges and Future Directions
In the realm of inorganic nanomaterials, the synthesis and characterization process present formidable challenges that demand meticulous attention. Section 5.1 delves into the current hurdles faced by researchers in the synthesis and accurate characterization of these materials. From issues related to reproducibility to complexities in controlling the size and morphology of nanoparticles, scientists grapple with multifaceted obstacles. Addressing these challenges is not merely an academic pursuit; it is a prerequisite for unlocking the full potential of inorganic nanomaterials in various applications.
Moving beyond the laboratory, Section 5.2 directs our attention to the ethical considerations and potential environmental impact associated with nanomaterials. The responsible development of these materials becomes imperative for a sustainable future. As the applications of inorganic nanomaterials expand across industries, questions of ethical usage, disposal, and long-term effects on ecosystems gain prominence. This section prompts a thoughtful exploration of the delicate balance between technological advancement and environmental responsibility, urging scientists and policymakers to navigate this frontier with caution and foresight.
In the final stretch of our exploration, Section 5.3 invites us to gaze into the future of inorganic nanomaterials. What lies ahead? Emerging trends, innovations, and potential breakthroughs are on the horizon. The section encourages us to envision a landscape where nanomaterials redefine their role in science and technology. Predictions, speculations, and insights into transformative developments in the field await, sparking curiosity about the next chapter in the evolution of inorganic nanomaterials.
Conclusion
Inorganic nanomaterials have emerged as transformative agents within the expansive realm of nanotechnology, heralding a new era of possibilities across diverse scientific and technological domains. This blog endeavors to traverse the fascinating landscape of inorganic nanomaterials, exploring their synthesis methods, unique properties, and the vast spectrum of applications that underscore their unprecedented potential.
The synthesis of inorganic nanomaterials represents a cornerstone of their burgeoning significance. Researchers employ various techniques, ranging from bottom-up approaches like chemical vapor deposition and sol-gel methods to top-down methodologies such as lithography and ball milling. Each method bestows distinct characteristics upon the resulting nanomaterial, influencing its size, shape, and composition. Understanding and mastering these synthesis techniques unlock the door to tailoring nanomaterials with precision—a key driver in harnessing their potential.
One of the defining features of inorganic nanomaterials is their unique properties at the nanoscale. Quantum effects, surface area enhancement, and size-dependent optical and electronic behaviors are just a few examples of the remarkable attributes exhibited by these materials. These properties often deviate significantly from their bulk counterparts, opening up novel avenues for exploration and exploitation. Nanomaterials, such as quantum dots, exhibit quantum confinement effects, enabling tunable electronic properties that find applications in areas like optoelectronics and bioimaging.
The applications of inorganic nanomaterials span an array of fields, promising breakthroughs in medicine, energy, electronics, and environmental remediation. In the biomedical realm, nanomaterials serve as targeted drug delivery vehicles and imaging agents, capitalizing on their size and surface properties to navigate biological systems effectively. In energy, nanomaterials contribute to the development of advanced batteries, efficient solar cells, and catalysts for clean energy production. The electronics industry benefits from nanomaterials' unique electronic properties, leading to the creation of faster and more efficient electronic devices.
Beyond their applications, inorganic nanomaterials play a pivotal role in addressing global challenges. Nanotechnology-enabled solutions for water purification, pollution control, and sustainable energy have the potential to revolutionize environmental remediation efforts.
In conclusion, the journey through the world of inorganic nanomaterials is a testament to their profound impact on the landscape of nanotechnology. From the intricacies of their synthesis to the manifestation of unique properties and the expansive realm of applications, these materials are shaping the future in ways previously unimaginable. As researchers continue to unlock their potential, the transformative power of inorganic nanomaterials is poised to redefine the boundaries of what is possible in science and technology.