Analyzing Spectroscopy Data in Inorganic Chemistry Assignments
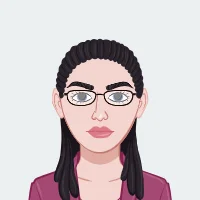
In the intricate landscape of inorganic chemistry assignments, the examination of spectroscopy data emerges as a fundamental cornerstone. Spectroscopy, a formidable tool in the realm of analytical chemistry, plays a pivotal role in unraveling the mysteries of molecular structures and compositions through the study of interactions between matter and electromagnetic radiation. This comprehensive guide endeavors to demystify the intricacies surrounding spectroscopy data analysis, serving as an invaluable resource for students and researchers alike, arming them with the knowledge to navigate through the complexities of their inorganic chemistry assignments with confidence and precision. If you need assistance with your inorganic chemistry assignment, I'm here to provide guidance and support to help you tackle spectroscopy data analysis and excel in your studies.
Spectroscopy, as a technique, encompasses a diverse array of methods such as infrared, ultraviolet-visible, nuclear magnetic resonance, and mass spectrometry, each offering a unique perspective into the properties of compounds. The elucidation of molecular structures, identification of functional groups, and determination of chemical compositions are among the myriad applications of spectroscopy. This guide aims to equip learners with a profound understanding of the principles underlying these techniques, enabling them to extract meaningful information from spectroscopic data with ease.
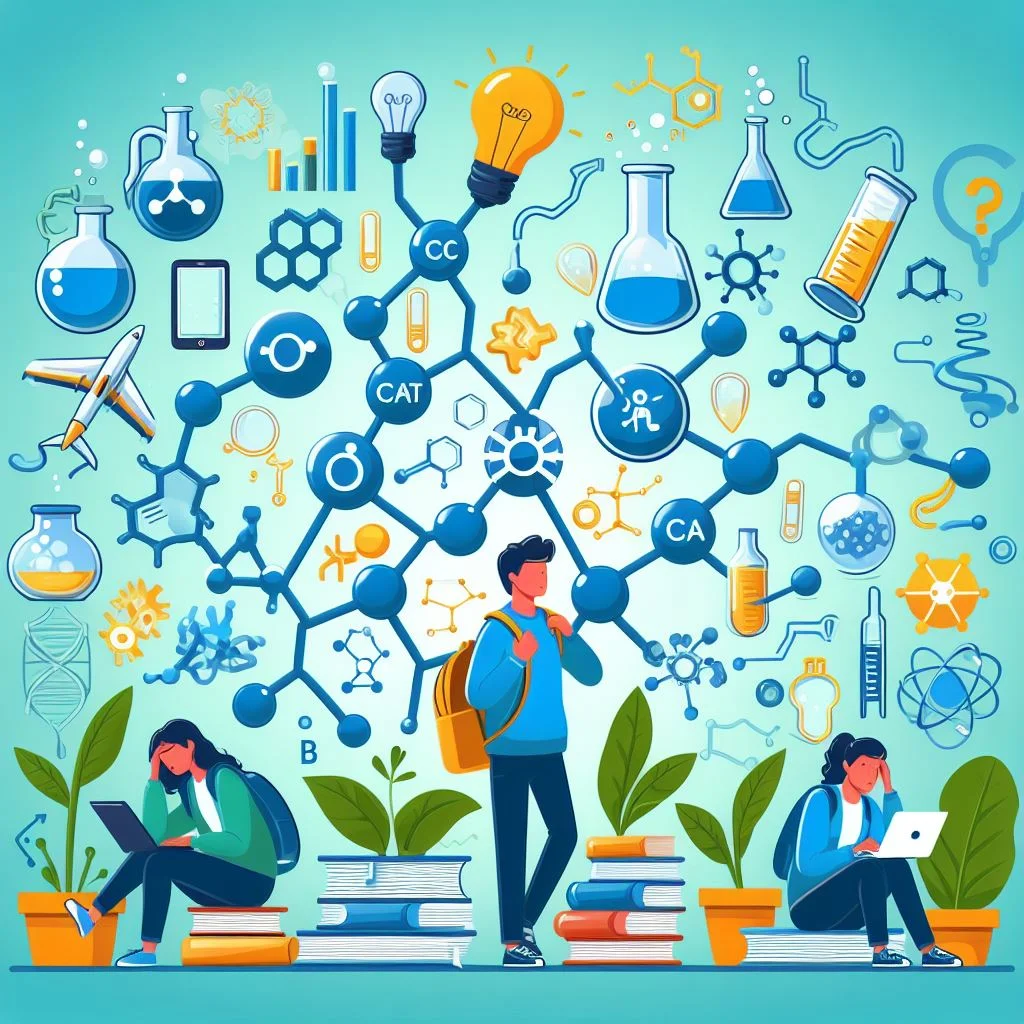
Understanding the intricacies of spectroscopy data analysis requires a systematic approach, and this guide provides a step-by-step elucidation of the process. From the acquisition of raw data to the interpretation of peaks and patterns, readers will be guided through the entire analytical journey. Moreover, practical tips and examples are interspersed throughout the guide to reinforce theoretical concepts, ensuring a holistic comprehension of the subject matter.
Empowering students and researchers, this guide instills a sense of confidence in tackling inorganic chemistry assignments. By offering a comprehensive roadmap through the multifaceted landscape of spectroscopy data analysis, it becomes a reliable companion for those seeking precision and clarity in their exploration of the fascinating world of inorganic chemistry. With this resource at their disposal, individuals are poised to not only comprehend the complexities of spectroscopy but also to apply this knowledge effectively in their academic and research pursuits.
Introduction to Spectroscopy in Inorganic Chemistry
Spectroscopy, a cornerstone in the realm of analytical chemistry, unfolds a diverse array of techniques that provide invaluable insights into molecular structures. In the domain of inorganic chemistry, several indispensable methods, including UV-Visible spectroscopy, infrared spectroscopy (IR), nuclear magnetic resonance (NMR), and X-ray crystallography, assume pivotal roles in unraveling the intricacies of chemical compositions. Delving into the principles underpinning these techniques is essential for adeptly deciphering the wealth of data they generate.
UV-Visible spectroscopy operates on the principle of molecular absorption of ultraviolet and visible light. This technique is particularly adept at revealing electronic transitions within molecules, shedding light on the arrangement of electrons and offering a window into the overall structure of compounds.
Infrared spectroscopy, on the other hand, focuses on the absorption of infrared radiation, elucidating the vibrational modes of molecular bonds. By analyzing the unique fingerprint regions of a spectrum, researchers can deduce the types of functional groups present in a compound, facilitating the identification and characterization of molecules.
Nuclear magnetic resonance spectroscopy relies on the magnetic properties of certain atomic nuclei, such as hydrogen. Through the application of strong magnetic fields and radiofrequency pulses, NMR provides detailed information about the local environment of nuclei within a molecule, allowing scientists to discern molecular structures with remarkable precision.
X-ray crystallography stands as a powerful technique for visualizing the three-dimensional arrangement of atoms in a crystal lattice. By directing X-rays through a crystal and analyzing the resulting diffraction pattern, researchers can construct detailed molecular models, unraveling the spatial relationships between atoms.
Understanding the fundamental principles behind these techniques empowers scientists to interpret the complex datasets generated by UV-Visible spectroscopy, infrared spectroscopy, nuclear magnetic resonance, and X-ray crystallography. This knowledge not only enhances the comprehension of molecular structures but also fuels advancements in various scientific disciplines, ranging from material science to pharmaceuticals, propelling the frontiers of inorganic chemistry.
UV-Visible Spectroscopy
UV-Visible spectroscopy is a crucial analytical technique in the realm of inorganic chemistry, as it delves into the absorption of ultraviolet and visible light by molecules. This method has proven invaluable for researchers seeking insights into electronic transitions and the bonding nature within inorganic compounds. The distinctive absorption patterns exhibited by these compounds in the UV-Visible range serve as fingerprints that enable scientists to deduce vital information about the molecular composition.
Infrared spectroscopy (IR) is another indispensable tool that focuses on the vibrational transitions of molecules. By studying the absorption of infrared radiation, researchers can identify functional groups, providing valuable clues about the molecular structures of inorganic compounds. In the realm of inorganic chemistry assignments, the interpretation of IR spectra is a common practice, allowing for a comprehensive understanding of compound composition.
Nuclear Magnetic Resonance (NMR) spectroscopy stands out as a technique that relies on the magnetic properties of atomic nuclei. Inorganic compounds showcase unique NMR spectra, offering intricate details about the local environment of nuclei within a molecule. Deciphering NMR data is essential for deducing molecular connectivity and conformation, playing a pivotal role in unraveling the intricacies of inorganic molecular structures.
X-ray Crystallography emerges as a powerful and indispensable technique employed by inorganic chemists for determining the three-dimensional structure of crystals. This method allows scientists to visualize the precise arrangement of atoms within a compound, providing unparalleled insights into complex structures. The ability to discern the spatial arrangement of atoms contributes significantly to the comprehensive characterization of inorganic compounds.
Strategies for Spectroscopy Data Analysis
In the realm of spectroscopy and analytical chemistry, several key techniques play a pivotal role in unraveling the mysteries of molecular structures and properties. One fundamental aspect is peak identification in UV-Visible and IR spectroscopy. These techniques rely on the absorption of light at specific wavelengths, with distinctive peaks corresponding to different functional groups or electronic transitions. A deep comprehension of these patterns is indispensable for accurate data interpretation, serving as the cornerstone for subsequent analyses.
Moving to NMR spectroscopy, a crucial skill is the integration of peaks. NMR spectra present peaks with varying intensities, and the process of integration provides valuable insights into the number of protons contributing to each peak. Mastery of integration is essential for determining molecular ratios, enabling researchers to make informed conclusions about the composition of a given sample. This proficiency is a linchpin in extracting meaningful data from NMR experiments.
Chemical shift analysis is another critical component in NMR spectroscopy. By scrutinizing the chemical shifts in spectra, chemists can glean information about the magnetic environment surrounding nuclei. This analytical prowess allows for deductions about neighboring atoms and functional groups, proving especially vital when dealing with intricate inorganic compounds.
In the realm of structural elucidation, X-ray crystallography takes center stage. Crystallographic refinement, a pivotal step in this process, involves adjusting atomic coordinates to align with experimental data. This meticulous refinement results in a precise three-dimensional representation of a crystal's structure. Understanding the principles of crystallographic refinement is imperative for obtaining accurate structural information, guiding researchers in comprehending the spatial arrangement of atoms within a crystal lattice.
Common Challenges in Spectroscopy Data Analysis
Spectroscopy, a powerful analytical technique, plays a crucial role in unraveling the composition of complex mixtures. However, navigating challenges such as overlapping peaks, low signal-to-noise ratios, and instrumental artifacts is paramount for accurate and meaningful data interpretation.
One common hurdle is the presence of overlapping peaks in spectroscopy data, particularly in complex mixtures. Resolving this issue requires the application of advanced deconvolution techniques and specialized software tools. These tools aid in untangling overlapping signals, enabling researchers to identify individual peaks and extract pertinent information. Without addressing this challenge, the risk of misinterpretation or oversight of important features in the spectrum is significant.
The signal-to-noise ratio (SNR) is another critical factor that influences the quality of spectroscopy data. Low SNR can obscure relevant details, making it challenging to distinguish true signals from background noise. To enhance signal quality and data reliability, practitioners often employ techniques such as signal averaging and optimize experimental conditions. By doing so, they amplify the signal while minimizing the impact of noise, ensuring a clearer and more accurate representation of the underlying chemical information.
Instrumental artifacts pose yet another hurdle, introducing inaccuracies that can compromise the integrity of spectroscopy data. Baseline drift and distortion are common issues that arise during measurements. Regular instrument calibration is imperative to correct and maintain accuracy. Additionally, meticulous data preprocessing, including baseline correction, helps mitigate the impact of instrumental artifacts. These measures are essential for ensuring the fidelity of the acquired data and facilitating precise analysis.
Advanced Techniques in Spectroscopy Data Analysis
Multidimensional Nuclear Magnetic Resonance (NMR) and computational methods are pivotal components in advancing the field of inorganic chemistry, offering researchers unparalleled insights into molecular structures and dynamics. These cutting-edge techniques have revolutionized the way scientists explore complex systems, providing enhanced spectral resolution and complementing experimental data with powerful computational tools.
Multidimensional NMR techniques stand out as a cornerstone in unraveling the intricate details of molecular structures. Traditional one-dimensional NMR spectra often face challenges in resolving overlapping signals, especially in intricate chemical environments. Enter multidimensional NMR, a paradigm shift that introduces additional dimensions to the spectra, offering a more comprehensive view of molecular interactions. By incorporating multiple dimensions, researchers can dissect complex systems with unprecedented precision. This enhanced spectral resolution is particularly beneficial when studying large biomolecules, catalysts, or other intricate chemical systems. Multidimensional NMR not only aids in identifying individual components but also provides valuable information about their spatial arrangement and dynamic behavior.
On the computational front, the advent of powerful tools in computational chemistry has propelled inorganic chemistry research into new realms. Quantum mechanical calculations and molecular dynamics simulations have become indispensable in the analysis of spectroscopy data. Computational methods complement experimental results by offering a theoretical framework to interpret complex spectra. Quantum calculations provide insights into electronic structures and energetics, shedding light on the underlying principles governing chemical interactions. Molecular dynamics simulations, on the other hand, offer a dynamic perspective, showcasing how molecules move and interact over time.
The synergy between experimental data and computational methods is a hallmark of contemporary inorganic chemistry research. Researchers can validate and refine their experimental findings using computational models, ensuring a more robust understanding of the studied systems. This integrated approach accelerates the discovery process and opens avenues for designing novel materials, catalysts, and pharmaceuticals.
Case Studies: Applying Spectroscopy Data Analysis in Research
Spectroscopy, a powerful analytical technique, finds practical application in various realms of inorganic chemistry. By delving into real-world case studies, we can unravel the intricate ways in which spectroscopy data analysis contributes to the advancement of scientific knowledge.
One compelling example lies in elucidating the coordination geometry of transition metal complexes. Spectroscopy enables researchers to study the interactions between transition metals and ligands by analyzing the absorption, emission, or scattering of light. Through this, intricate details about the spatial arrangement of atoms within these complexes are unveiled. This knowledge is invaluable in designing catalysts for chemical reactions, understanding electronic structures, and exploring the reactivity of these complexes.
Another noteworthy application of spectroscopy is in identifying unknown compounds through spectral matching. In this case, researchers utilize the unique spectral fingerprints of compounds to determine their composition. By comparing experimental spectra with reference spectra, scientists can pinpoint the identity of unknown substances. This capability is particularly crucial in environmental analysis, forensics, and pharmaceutical research where the identification of compounds can have profound implications.
The significance of spectroscopy becomes evident across diverse inorganic chemistry research areas. Whether unraveling the mysteries of transition metal complexes or solving the puzzle of unknown compounds, spectroscopy serves as a versatile tool. Its ability to provide detailed molecular information, coupled with advancements in technology, has expanded its applications beyond traditional boundaries.
These case studies emphasize the pivotal role of spectroscopy in advancing our understanding of inorganic chemistry. As researchers continue to push the boundaries of scientific exploration, spectroscopy stands as a steadfast ally, offering insights into the molecular intricacies that govern chemical phenomena. The marriage of theoretical knowledge and practical applications showcased in these studies highlights spectroscopy as a cornerstone in the edifice of inorganic chemistry research.
Conclusion:
Mastering the intricate realm of spectroscopy data analysis is undeniably paramount for triumph in the realm of inorganic chemistry assignments and research. This multifaceted skill set serves as a linchpin, enabling scholars to decipher the intricate language embedded within chemical spectra. As a dynamic field within the broader spectrum of analytical chemistry, spectroscopy plays a pivotal role in unraveling the molecular intricacies of inorganic compounds.
A foundational aspect of this mastery lies in comprehending the underlying principles governing each spectroscopic technique. Whether delving into the nuances of UV-Visible, infrared, or nuclear magnetic resonance (NMR) spectroscopy, a profound understanding of the fundamental principles is indispensable. Each technique offers a unique perspective, unveiling specific details about the molecular structure, bonding, and composition of inorganic substances. Armed with this knowledge, students and researchers gain the power to interpret complex spectra with precision.
Equally vital is the adoption of effective data analysis strategies. Spectroscopy generates vast amounts of data, and extracting meaningful insights requires a judicious approach. Employing mathematical algorithms, statistical tools, and computational methods, researchers can discern patterns, identify peaks, and correlate spectral features to specific molecular attributes. Proficiency in these analytical techniques not only enhances the accuracy of results but also empowers scientists to draw robust conclusions from their experiments.
Moreover, staying abreast of advanced methodologies is imperative in a field characterized by rapid technological evolution. Emerging techniques, such as mass spectrometry coupled with spectroscopy or advanced computational methods, present new avenues for exploration. A proactive approach to incorporating these innovations into research methodologies ensures that scholars remain at the forefront of inorganic chemistry.